24.07.2018
The High-Resolution Microcalorimeter X-ray Imaging Rocket
Micro-X is an X-ray space telescope payload being developed for NASA's sounding rocket program. We will fly the first Transition-Edge Sensor (TES) microcalorimeter array in space. Our versatile science program includes high-resolution spectroscopy of supernova remnants and searches for dark matter in the Milky Way.
-
CHICAGO (CBS) — It’s an out of this world project 10 years in the making for a group of Northwestern researchers.
A NASA-funded rocket called Micro-X launched Sunday from New Mexico.
Led by a Northwestern University research team, the rocket will provide detailed x-ray images of objects in space.
This is the first time this type of instrument has been used in space exploration.
MICRO-X HARDWARE
Rocket
We've been hard at work fabricating and assembling hardware for our upcoming flight. The rocket is pictured above and to the side. The science instrument, including the mirror, optical bench, cryogenic cooling system, detectors and readout electronics will be carried by a Terrier/Black Brant IX sounding rocket. The rocket will also house attitude control, boost guidance, recovery, and telemetry systems.
Mirror and Optical Bench
The Micro-X mirror is a conically approximated Wolter I optic recycled from the previous Supernova X-ray Spectrometer (SXS) sounding rocket flight in 1988. It has been refurbished and aligned at Goddard Space Flight Center. The optical bench is a rigid aluminum structure that holds the mirror such that its focal point is aligned with the detector array.
ADR
The Micro-X detectors are run at ~50 mK to limit the thermal noise in the signal. These are cooled and temperature controlled using an adiabatic demagnetization refrigerator (ADR). This uses the cycling of a superconducting magnet (cooled by liquid helium) to cool a paramagnetic Ferric Ammonium Alum (FAA) salt. This salt is connected to the detector array through a magnesium cold finger. Our detectors are superconductors held at the superconducting transition (see TES's for more information on the detectors). The temperature at which a material becomes superconducting is affected by the magnetic field it sees. We therefore need to protect our detectors from magnetic fields. A niobium magnatic shield and the superconducting magnet's bucking coil combine to provide this protection.
After everything has been cooled to ~1.8 K by filling the helium can with liquid helium and pumping on the bath, the superconducting magnet is ramped up to approximately 4 T. This aligns the spins of the atoms in the salt. The salt is thermally connected to the rest of the system during this process so it cools to ~1.8 K. Next, the thermal connection is removed and the magnet is ramped down slowly to zero magnetic field. This causes the spins in the salt to randomize, raising the entropy. Since this process is adiabatic the temperature responds to the rise in entropy by dropping. In practice the magnet is not ramped down all the way at the end of the cycle. As heat flows into the salt from the detector stage we continue to ramp down to maintain the temperature of the salt. The expected base temperature for our system is on the order of 50 mK.
The magnet, salt, magnetic shields, FEA and liquid helium stage boards are all part of an assembly called the 'insert' that lifts out of the flight ADR as a whole. This is designed to expedite repairs and improve ease of access. The insert sits on the helium can. Infrared filters (silicon meshes with very thin layers of polyimide and aluminum deposited on them) protect the detectors from infrared radiation from warmer stages in the refrigerator. Another possible cause of detector heating is vibration. Rocket launch produces a large spectrum of vibrations and if the physical system is vibrated at resonance a significant amount of dissipation can occur raising the temperature of the cold stage significantly. The forward and aft damping assemblies are designed to minimize detector heating through vibration.
The science instrument, including the ADR, is being commissioned at Northwestern. NASA Goddard has developed the detectors, and NIST (Boulder, CO) will provide the readout electronics. The image below shows the assembled ADR with radiation shields removed. The outer red vacuum enclosure is 16 inches across.
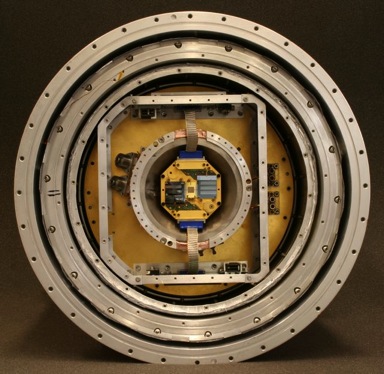

FEA
The focal plane is enclosed in a box that thermally shields the detectors. This assembly is called the Front End Assembly (FEA). This includes the detector array, the first two superconducting amplification stages, two germanium resistance thermometers (GRTs) and interface chips. The superconducting amplification stages are made up of Superconducting Quantum Interference Devices (SQUIDs). The FEA is assembled with a detector array and interface chips. It is shown below without the lid and with the lid and connectors attached. Missing from the assembly in these photographs are the GRTs and infrared filters.

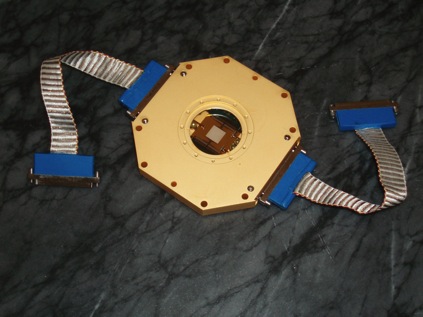
Science
Figure 1 (left): Chandra false-color of Cas A from a mega-second observation. The image is overlaid with the Micro-X pixel array outline (indicating the Micro-X field of view), and a 2.4' PSF circle (indicating the Micro-X target energy resolution).
Figure 2 (right): Simulated Micro-X 300 s observation, There are 12,998 total integrated counts. The color bar indicates the total counts per pixel, and the angular resolution is shown in the PSF cirlce The colorbar scales linearly with event count.
The Future of X-ray Astronomy
The Micro-X mission is a rocket-borne telescope with a new type of X-ray detector that will revolutionize X-ray astrophysics and the use of high resolution X-ray spectroscopy. Our detectors, called Transition-Edge Sensor (TES) Microcalorimeters, measure the energy of a photon by sensing the small change in temperature when the photon is absorbed in the TES. With TESs, the combination of high energy resolution, high efficiency, precise timing, and potential for true imaging spectroscopy at X-ray energies is unparalleled by any other technology today. TESs are being developed for future NASA missions like IXO, and will open up new frontiers in our ability to study black holes and strong gravity, dark matter, dark energy, the evolution of structure formation in our universe and the cycles of matter and energy.
Micro-X stands to advance the science of spaceborne X-ray detectors and, with the first flight, the understanding of the complex Cassiopeia A remnant.
Micro-X will be a multi-flight program. Future flights may study a varied set of astrophysical problems, among them the physics of the cores of clusters of galaxies, and the physics of accretion, jets, and outflows in neutron stars and black holes in bright X-ray binaries.
These are the specifications for the Micro-X telescope and detector:
Energy resolution | <4 eV at 1 keV |
Effective area | 300 cm2 at 1 keV |
Half power diameter | 2.6 arcmin |
Focal length | 2100 mm |
Field of view | 11.8 arcmin |
Detector array | 128 pixel (quasi-circular) |
Pixel size | 600 μm (0.8 arcmin) |
Bandpass | 0.2 - 3.0 keV |
+++
THE SCIENCE OF TES'S
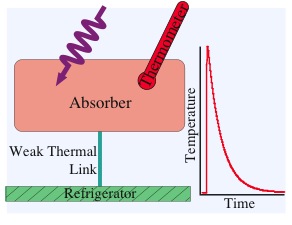
The idea behind a microcalorimeter is deceptively simple: it takes advantage of the unique properties of a superconductor to make an ultra-sensitive thermometer. A superconductor transitions from the normal state (with finite resistance) to the superconducting state (with zero resistance) over a small span of about 1 mK, which makes for a sharp 'edge'. A Transition-Edge Sensor (TES), as the name suggests, is a superconducting film held in this transition. Since the edge is sharp, a tiny change in temperature that this TES sees - such as a photon being absorbed - translates to a big change in resistance, from which the energy of the original photon can be extracted.
The execution of the idea, however, requires some electronic and cryogenic ingenuity. An absorber, which provides more material to stop the energetic photons, is cooled along with the TES to the transition temperature of the TES, typically around 100 mK. (Low-temperature superconductors are used because the intrinsic thermodynamic fluctuations are lower in the absorber at such temperatures.) When a photon is absorbed, its energy is converted into thermal energy which heats up the absorber by a small amount (typically less than 1 mK). The system cools down again quickly through negative electrothermal feedback and its connection to the refrigerator. An array of SQUID electronics monitors the changing current at constant voltage through the TES as the absorber temperature changes. The integral of this current pulse is proportional to the energy of the photon, and the time of arrival is determined from the rise of the pulse. For more info on microcalorimeters, follow this link.
The Micro-X focal plane will consist of a 12x12 square array of these absorber-TES pairs. Such fine-tuned thermometry will allow for precision spectroscopy of astrophysical sources.
The Micro-X focal plane array, 7.2 mm across
An image of the array from beneath, showing the absorber supports and the location of the TES
+++
THE MICRO-X TARGET: THE CASSIOPEIA A SUPERNOVA REMNANT
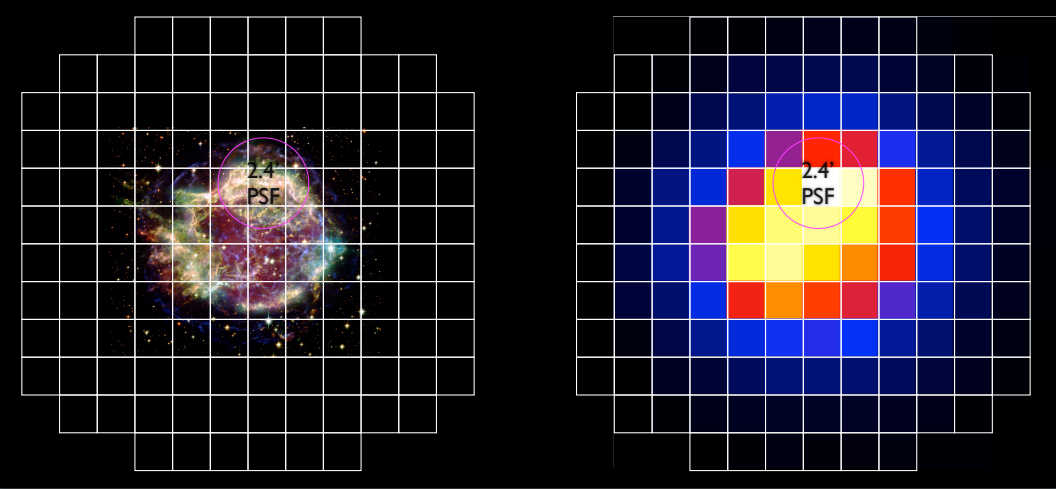
Cassiopeia A (Cas A) is a target well-suited to studying the physical processes that occur in core-collapse Supernova Remnants (SNRs).It is a very bright target located at a well-constrained distance of 3.4 kpc. It is the product of a Type IIb supernova, and at ~340 years old, it is the second youngest known SNR in our galaxy.
The brightest emission in Cas A is within the radius where stellar ejecta are illuminated after being pushed outward, then compressed and heated by the reverse shock, forming the 200" diameter "Bright Ring". Iron-rich ejecta are unexpectedly observed futher out from the center than silicon-rich ejecta; this may be an overturning of ejecta layers in the explosion because heavier elements are formed in the very core of the supernova. Figure 1 shows a Chandra observation of Cas A's expanding shell of hot gas.
The physical conditions of the SNR are studied by determining the ionization state of the plasma. At X-ray energies, this means studying the line fluxes of the hydrogen- and helium-like emission from abundant elements (e.g., Si, Mg, and the range of Fe ions from Fe XVII to Fe XXVI). The Si XIII triplet (~1.85 keV) will be the strongest emission line complex seen by Micro-X.
Micro-X's high-resolution observation of Cas A will allow the study of previously-entangled emission lines for the first time. Micro-X simulations project ~13,000 counts incident upon the array in a 300 second observation, as shown in Figure 2. The resulting simulated spectrum is shown in Figure 3.
Micro-X will be the first instrument to test if the plasma states are the same for Fe- and Si-rich ejecta by disentangling the Fe-L forest and Ne emission between ~1-1.5 keV. This analysis cannot be done with either Chandra ACIS (low spectral resolution) or Chandra HETG.
Ni and Fe are particularly important for understanding nucleosynthesis during the supernova. In the outer regions of the SNR, Ni-L emission may be observed for the first time, disentangled from the abundant Fe-L emission in the outer region. The Micro-X spectrum will be used with the Chandra ACIS and HETG spectra to model abundance, ionization, and Doppler structures to obtain average plasma conditions for several parts of the remnant.
Micro-X will also help to solve the "oxygen problem" in SNRs. Cas A is an oxygen-rich SNR, with strong strong oxygen emission at optical wavelengths. The actual amount of oxygen in Cas A is an unknown due to absorption and the limited energy resolution of CCD detectors. The presence of an oxygen-driven continuum in the X-ray emission spectrum is a point of debate, and Micro-X will help to solve this issue by constraining the amount of oxygen in Cas A through its measurement of O VII and O VIII lines, as shown in Figure 3.
The goals for the Micro-X observation of Cas A are to:
- Determine the line flux and associated temperature of observed Fe-L emission
- Perform plasma diagnostics and measure velocities of discrete ejecta knots
- Provide a global elemental assay for Cas A
- Measure the dynamic width of the Bright Ring
Cas A is a complex object with structure on multiple spatial scales and typical knot sizes on a 1" scale. Micro-X will observe emission from multiple ejecta, each with their own Doppler-shifted velocity. It will only be sensitive to the brightest knots and emission lines in a single flight. The brightest lines are expected to produce 10's of counts in a single observation, providing the statistics for a velocity resolution of ~150 km/s. At least 5 pixels in the array are projected to achieve statistically strong kinematic results (with very good signal-to-noise and clear Doppler structure). In addition to measuring individual ejecta knot Doppler velocities, Micro-X will measure the dynamical width of the Bright Ring from the assortment of low-level emission that broadens spectral lines. This Micro-X result from observations of high temperature (T = 107K) gas will be compared to a Spitzer spectrum showing a dynamical width of ~1000 km/s for the comparatively low (T = 104K) un-decelerated ejecta comprising the Bright Ring. This comparison will determine how strong of an effect post-shock deceleration and turbulence are on X-ray-emitting ejecta.
Figure 3: Simulated global spectrum of Cas A from co-adding all pixels. The high resolution observation will allow sensitivity to new physics.
+++
THE SCIENCE OF PUPPIS A
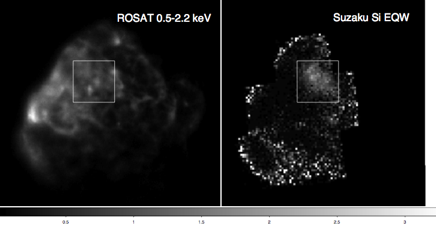
A future Micro-X observation target is the Silicon Knot region of the Puppis A supernova remnant. Puppis A is a bright, middle-aged Galactic supernova remnant, the remnant of a star that exploded approximately 4,000 years ago. Its ejecta is plowing into the surrounding circumstellar medium, which shocks the ejecta and creates a hot (> 106 K) plasma. This plasma emits a rich X-ray spectrum full of lines from atomic transitions which encode the state, composition, and temperature of the plasma. A high energy resolution observation is needed to unravel this complex spectrum. The Silicon Knot (Si Knot) region of Puppis A is particularly interesting because much of the silicon is explosively synthesized.
The improved sensitivity and spectral resolution of the CCDs on the Suzaku X-ray Observatory have led to the identification of a coherent, large-scale ejecta feature in "equivalent width", or line/continuum images, of Si emission in Puppis A. This roughly 8 x 10 arcminute region is located in the north/northeast region of the remnant, somewhat farther out in radius than the optically-identified O ejecta.
The Suzaku observations show that the large ejecta knot can be subdivided into two parts, with the northern half dominated more strongly by Mg and Si than the southern half, in which the O and Ne emission are relatively stronger. The element abundance maps obtained by Suzaku confirm that this entire feature is enhanced not only in Si, but also in O, Ne, Mg, and possibly Fe, all of which have strong lines that will be accessible to Micro-X.
The goals of our Micro-X observation of the Si ejecta knot in Puppis A are to:
- Search for velocity shifts in the various emission lines and measure the line structures to obtain information about the dynamics of the various ejecta elements.
Velocity and dynamics will give us direct information as to how well-mixed the ejecta are, and in particular, whether there were any differences in the dynamics of the outlying O and Ne ejecta compared to the inner Si and Fe ejecta. Although velocity shift measurements have been attempted with CCD spectra, those moderate resolution spectra do not resolve individual lines so that the energy shift of any particular line is partly obscured by the presence or absence of weak lines in the blend.
- Perform plasma diagnostics for the various emission lines of individual elements to ascertain how similar or different the thermodynamic states of the various elements are.
The progress to ionization equilibrium depends on both the density of the gas and the shock time. Complexities in the temperature structure are also expected, as a range of temperatures is indicated from Chandra, XMM-Newton, and Suzaku observations. In the case of the hard-to-see Fe L lines, the mere identification of the L lines in the spectrum gives a diagnostic of the range of temperatures in the gas.
- Refine estimates of element abundances in the ejecta.
Finally, once the dynamical effects and the physical properties of the emitting gas are understood, the high resolution spectrum from Micro-X will provide the means to refine estimates of element abundances in the ejecta. The detailed insights into the temperature and ionization structure are crucial to such an effort. Although the ejecta knot is only a relatively small part of the total ejected material, its large physical scale suggests that it may be a fair representation of the whole. The Micro-X observation would then enable an extremely detailed comparison to nucleosynthesis models for this remnant because it would involve a significant mass of a range of elements.
Simulated 300 second observation of Puppis-A by Micro-X (note logarithmic scale). Micro-X's high resolution clearly separates individual spectral lines, enabling detailed plasma diagnostics.
Quelle: Micro-X Collaboration