.
29.10.2015
COMETWATCH FROM EARTH: PEAK BRIGHTNESS AND COMA GASES
The latest update from the professional ground-based observing campaign of Comet 67P/Churyumov-Gerasimenko, with inputs from astronomers Colin Snodgrass, Alan Fitzsimmons, Cyrielle Opitom and Emmanuel Jehin.
While Rosetta made a far excursion 1500 km from the comet to get a view of the wider coma and plasma environment during late September/early October, Earth-based observers have also been continuing to monitor the comet – from even greater distances!
The image below was taken on 30 September, the same day that Rosetta reached its furthest distance from the comet during this excursion. To help put things into perspective, the image is also shown with two red dots: the right hand dot marks the centre of the nucleus, while the left hand one lies roughly 1500 km in the sunward direction, showing approximately where Rosetta was at the time the ground-based observations were made.
.
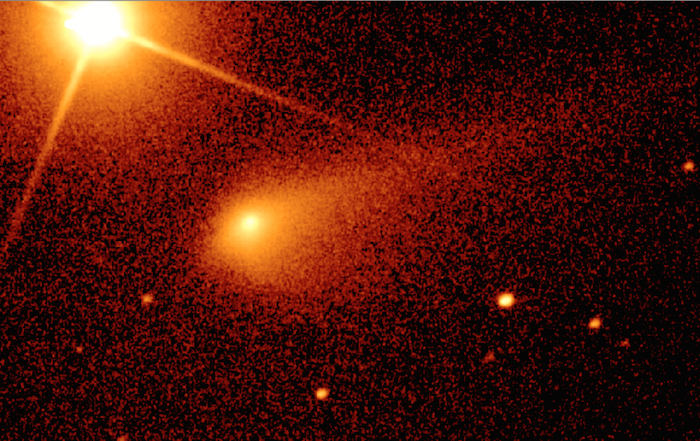
.
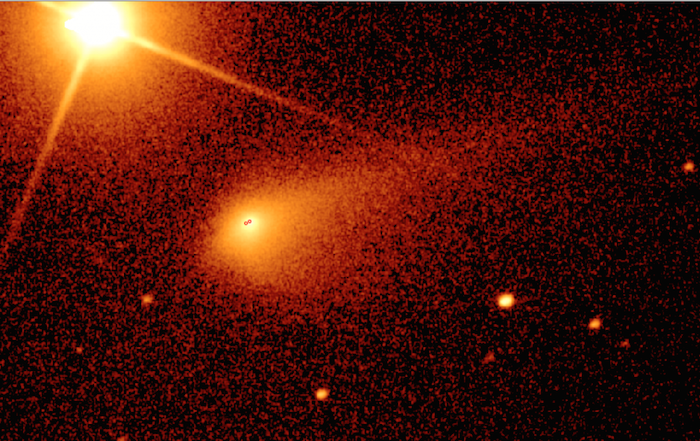
Caption: 20 second R-band exposure of Comet 67P/C-G using the Liverpool Telescope on 30 September 2015. The bottom image has been marked with two red dots to indicate the position of the nucleus and Rosetta, which was separated from the nucleus by 1500 km around this time. The comet was about 205 million km from the Sun on 30 September. The field of view is approximately 4.5 x 2.8 arcminutes, or 270,000 x 170,000 km. Credits: Alan Fitzsimmons / Liverpool Telescope
-
Peak brightness
Meanwhile, the ground-based astronomers have been analysing the comet’s brightness following perihelion – the comet’s closest approach to the Sun along its orbit – on 13 August. Based on measurements made by the 60-cm diameter TRAPPIST telescope located at the La Silla observatory in Chile, the comet appeared to show the peak in brightness at the end of August. Indeed, data obtained by the TRAPPIST telescope on 31 August indicated a dust production rate at the nucleus corresponding to approximately 1000 kg per second. The peak brightness on the same day was recorded as magnitude 12 (roughly 250 times dimmer than the faintest stars visible to the unaided naked eye, and thus decent-sized telescopes are needed to study the comet).
Since the peak dust production rate measured on 31 August, the overall activity has reportedly been declining steadily, following the trend anticipated from observations made of 67P/C-G during previous perihelion passages (see Snodgrass et al 2013).
.
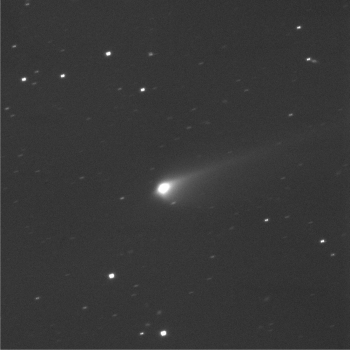
Comet 67P/C-G captured by the 2 m Liverpool Telescope at around 05:30 UT on 8 October 2015. The image is a combination of 9 x 15 second exposures, and shows the tail extending 400,000 km from the comet. On 8 October the comet was was roughly 270 million km from the Earth and 212 million km from the Sun. This image shows the full field of view of the IO:O camera on the Liverpool Telescope, 10.4 arcminutes on a side, or approximately 640,000 km at the distance of the comet. Credit: Colin Snodgrass / Liverpool Telescope
.
How much mass is the comet losing?
Using the 1000 kg/s dust loss rate, and knowing a few basic parameters of the comet’s characteristics, we can make some back-of-the-envelope estimates as to the amount of the comet’s surface that was being removed at this maximum. The calculation assumes that the density of the comet is around 500 kg/m^3, and considers the surface area of an idealised 4 km diameter sphere (5.0 x 10^7 square metres).
Based on Rosetta’s pre-perihelion measurements that indicate the dust:gas ratio was approximately 4 , that means roughly 80% of the material being lost is dust, with the rest dominated by water, CO, and CO2 ices. (Note: at the time of that blog post an estimate of 3 was made for perihelion, but the actual data has yet to be analysed.)
In any case, using 3 and 4 respectively, the total mass loss rate at its peak is likely in the range of about 100,000–115,000 tonnes per day.
Of course, that’s not a huge amount compared to the comet’s overall mass of around 10 billion tonnes. But nevertheless, a very simple calculation reveals that if, for example, the comet lost that much mass continuously for 100 days, it would correspond to roughly 0.4-0.5 metres of its surface being removed in that time.
Once Rosetta has returned to closer distances then more detail of how the comet’s surface has changed post-perihelion will be revealed.
.
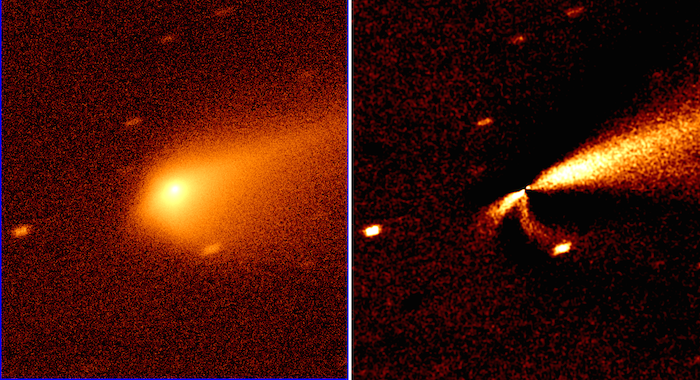
Comet 67P/C-G as seen by the Liverpool Telescope on 8 October. The Sun lies to the left of the comet, and the effects solar radiation pressure can clearly be seen driving the dust away, some of it to form the main tail to the right. Right-hand image: Processing to remove the symmetric part of the coma profile makes it possible to see the asymmetric structures in the denser region of the coma, including a southward excess of dust being bent back by the solar radiation pressure. Credit: Alan Fitzsimmons / Colin Snodgrass / Liverpool Telescope
.
Back to the comet’s coma, and from Rosetta’s close-up vantage point, several outburst events and hundreds of jets have been observed coming from the nucleus of 67P/C-G. It is not possible to discern these features directly from ground-based observatories, but processing of these images reveal clear asymmetries in the coma (see image above). Combining the big-picture views obtained by ground-based telescopes with Rosetta’s close-up study of individual jets and outbursts will help scientists understand the processes at work on the comet’s surface during these active periods, and how they are linked into evolution of the coma on the larger scales.
Coma gases
As well as the brightness and structures in the coma, astronomers are also busy analysing the chemical composition of the coma gases. These gases are illuminated by sunlight and glow at different wavelengths depending on their chemical make-up, allowing them to be identified and measured using a spectrograph, which splits light into its constituent wavelengths (as can be seen with a prism).
Now that the comet is relatively bright, many spectrograph-equipped telescopes are being used to measure the composition of the coma and the relative production rates of gases.
The LOTUS spectrograph was designed, built, and commissioned on the Liverpool Telescope in only six months, specifically for observations of Comet 67P/C-G. The previous spectrographs on the telescope were optimised for observations at red wavelengths, but the brightest gases in cometary comae emit most of their light at the blue-to-green end of the spectrum: this is why images of bright comets have a blue–green glow.
One of those gases regularly monitored by LOTUS is the cyano-radical CN, often referred to as cyanogen, the name for its molecular form (CN2). CN is a toxic gas and fluoresces at blue and near-UV wavelengths, as well as in the red. CN in the coma of a comet is thought to be produced when the toxic gas hydrogen cyanide (HCN) is dissociated (broken apart) by sunlight, and it is one of the most-widely studied gases in comets, giving some measure of the changing levels of activity.
Other gases commonly seen in the comae of comets include the CH, C3, and C2 radicals, the latter glowing at green wavelengths. Another important component is the OH radical, produced when solar ultraviolet photons split water molecules, of which comets have plenty.
OH glows in the near-UV and can be hard to study from Earth, because ozone in our atmosphere absorbs at these wavelengths. That’s a good thing for humans, as UV light is harmful, but makes it difficult to observe the OH emission from comets with ground-based telescopes.
Observations of OH from Comet 67P/C-G have been doubly challenging, as its apparent proximity to the Sun in the sky meant that it could only be studied in twilight, close to the horizon. The extra path length through more of the atmosphere led to even more absorption.
Fortunately, on the same mountain as the Liverpool Telescope, astronomers had access to the larger, 4.2-m diameter William Herschel Telescope (WHT). While the WHT has been surpassed in collecting area by the new generation of 8-m class telescopes, it has some unique strengths, not least its very sensitive spectrograph ISIS, optimised for observations and blue and near-UV wavelengths.
.
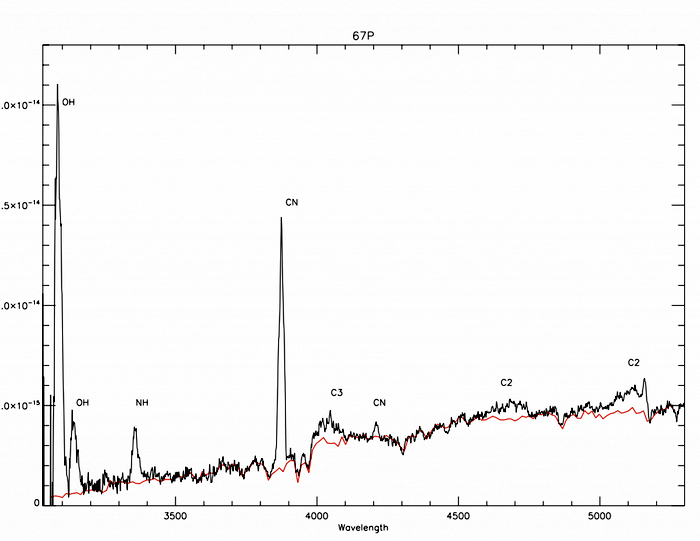
10 minute spectrum of Comet 67P/C-G using the William Herschel Telescope on 20 August 2015. The red line shows the part of the spectrum due to sunlight reflected by the dust grains in the coma. The spectrum has been corrected for the wavelength-dependent absorption caused by the atmosphere and for instrumental effects. Credits: Alan Fitzsimmons / WHT
This makes the WHT an ideal telescope for studying comets, and thus it was used to observe 67P/C-G in the early morning twilight of 20 August and again on 2 September. Even though the atmosphere transmitted only 3% of the UV light coming from OH molecules, there was enough water being released by the comet and therefore OH being produced that ISIS was able to make a clear detection of this gas.
These measurements allowed astronomers to calculate the amount of water being released by the comet in mid-August, yielding 90 kg per second. By comparison, Rosetta’s MIRO instrument measured up to 300 kg of water being released per second around perihelion.
Given the variability in the amount of gas being released from the comet at any given moment and uncertainties in the physical models applied to both the in situ and ground-based data, this is considered pretty close! As both sets of data are further analysed and the models refined, these numbers will likely move even closer.
.
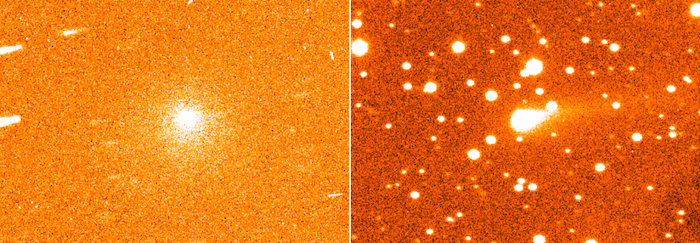
CN emission (left) and dust emission (right) of Comet 67P/C-G on 22 August 2015 with the TRAPPIST telescope. The image is 4.3' x 8.7', or 340,000 x 700,000 km. Credit: Cyrielle Opitom / Emmanuel Jehin / TRAPPIST.
And finally, what about the CN gas? Through a quirk of quantum physics, it is much better at absorbing and re-emitting light from the Sun than OH. So even though the CN emission from 67P/C-G was almost as bright as the OH emission, it only required the release of a mere 0.13 kg of HCN per second from the nucleus.
These ground-based observations of the constituents of the coma of 67P/C-G continue to give vital complementary information to that being obtained from the unique vantage point of Rosetta.
Quelle: ESA
---
Update: 31.10.2015
.
COMETWATCH 26 OCTOBER – 6 HOURS APART
CometWatch this week comprises two NAVCAM images acquired six hours apart on 26 October 2015.
The images have been lightly enhanced to reveal some detail of the comet’s activity (the unprocessed images are available at the end of the post). In the six hours that passed Rosetta also moved 2.3 kilometres closer to the comet, resulting in slightly different image scales between the two images.
.
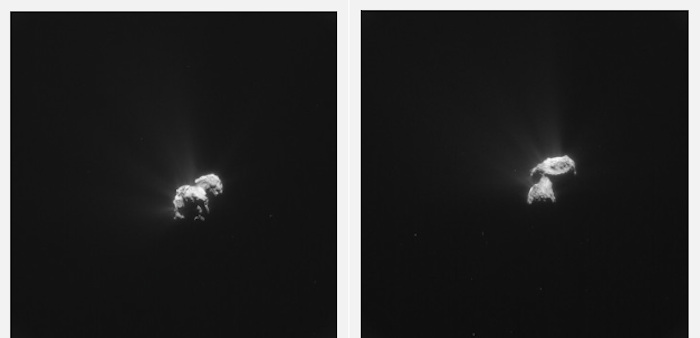
Caption: Left: Comet 67P/C-G at 13:31:01 UT on 26 October from a distance of 312.7 km. The image scale is 26.6 m/pixel and the image measures 27.3 km across. Right: The view 6 hours later, at 19:27:53 UT, from a distance of 310.4 km. The image scale for the second image is 26.5 m/pixel and the image measures 27.1 km.
-
Since Comet 67P/Churyumov-Gerasimenko’s rotation period is a little over 12 hours, these two images are separated by about half a comet rotation, offering complementary views of the nucleus. In both orientations, jets can be seen rising from the sunlit side of the nucleus.
In the left-hand image the large lobe is in the foreground, with Imhotep facing the viewer, albeit largely in shadow. Similarly, the outline of the Aten depression, also in shadow, can just be made out to the right. Above Aten, towards the centre of the image, are the Khepry and Aker regions, with Anhur towards the upper left of the large lobe.
On the small lobe in the background it is mostly Bastet that is visible, with a hint of Wosret appearing as the flat portion at the top left.
Six hours later and the comet’s small lobe is in the foreground, the large lobe in the background, with the Anuket region of the comet’s neck visible in between. On the large lobe the ridge separating Seth (itself not visible) from the smooth Anubis region can be readily picked out, appearing as the arc-shaped feature on the lower right edge of the large lobe; Seth is in fact beneath it, cast in shadow. The more rugged Atum and parts of Khonsu can be seen beyond, in the upper part of the image.
Meanwhile the rough terrain on the small lobe contains parts of Serqet, Nut, Ma’at and Maftet, with Wosret to the far left.
If you’re finding it hard to navigate, then do check out our interactive comet viewer alongside our CometWatch posts and toggle the regions on and off.
In other CometWatch news, 1003 images were added into the NAVCAM Archive Image Browser yesterday. They cover the period 11 March–5 May 2015 and include images from the 14 km close flyby on 28 March. Enjoy!
The two original 1024 x 1024 images from today’s post are provided below:
.
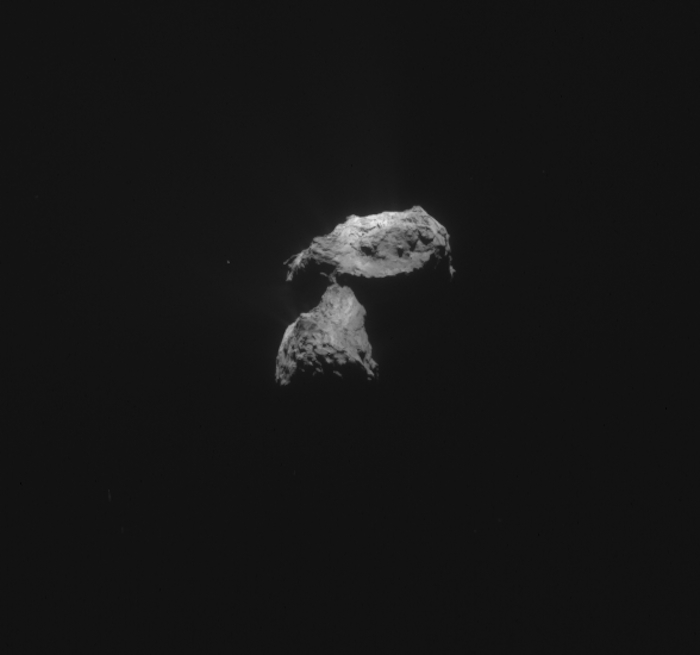
.
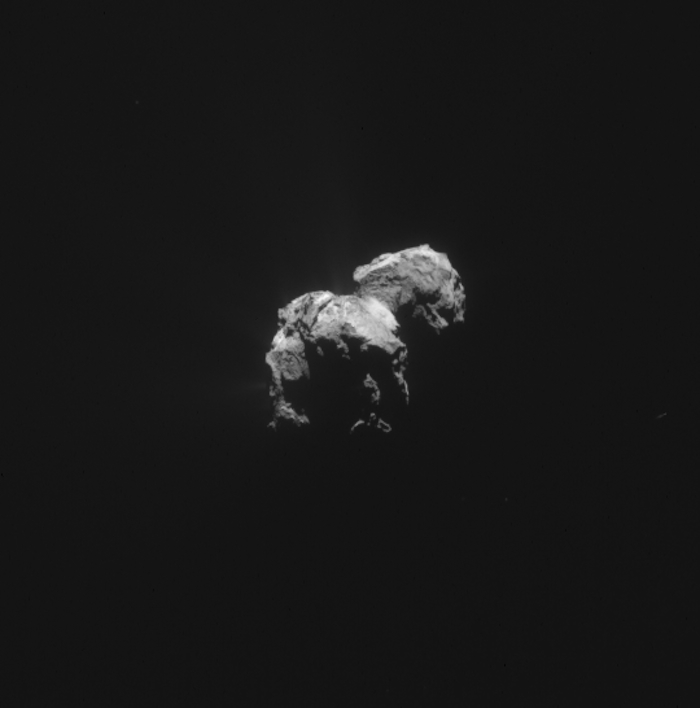
Quelle: ESA
---
Update: 3.11.2015
.
ASTRONOMY & ASTROPHYSICS: ROSETTA SPECIAL ISSUE
A special issue of the journal Astronomy & Astrophysics was published online on Friday, featuring 46 articles based on data collected by Rosetta and Philae in the mission's first year at Comet 67P/Churyumov-Gerasimenko. Topics covered include the comet's shape and formation theories, the evolution and nature of its activity, the composition of the coma, and the coma's interaction with the solar wind.
For a full list of contents,
http://www.aanda.org/articles/aa/abs/2015/11/contents/contents.html
.
Many of these papers were published over the past few months, and several were already covered by news articles here on the ESA blog and/or the main ESA web portal; you can find the news items linked from the table of contents by clicking on the ‘press release’ button.
Note that the papers are currently open access (this week) but require a free registration, as indicated here.
---
COMETWATCH FROM GAIA
This blog post is based on an image release published on the ESA Space Science portal .
.
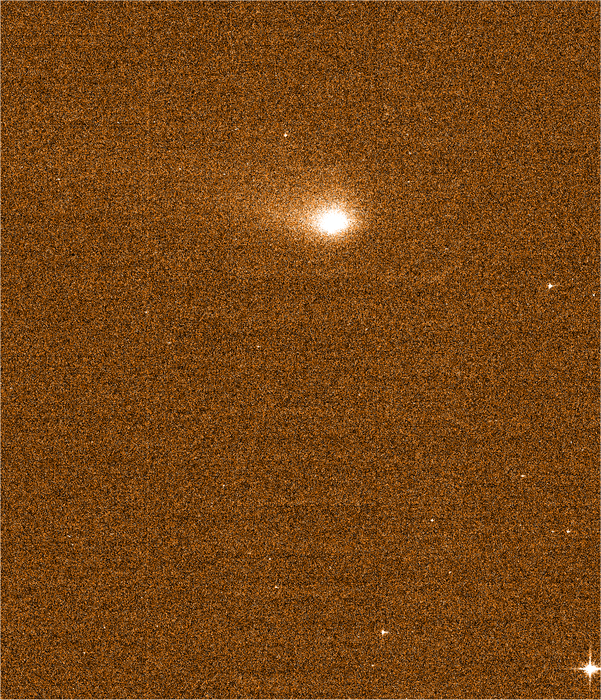
A Gaia image of Comet 67P/Churyumov–Gerasimenko, obtained on 14 September 2015.
At that time, the ESA Rosetta spacecraft was about 300 km from the nucleus of the comet, while Gaia was over 260 million kilometres away. The comet had reached the closest point to the Sun on its orbit about a month earlier, on 13 August.
The image shows the comet’s coma and tail. The nucleus and Rosetta, which was some 300 km from the surface at the time, are both hidden in the innermost pixel. A number of background stars are also sprinkled around the image, which measures about 4.5 arc minutes across – about one-seventh of the Moon’s diameter.
.
On 14 September 2015, Comet 67P/Churyumov Gerasimenko was imaged by Gaia, ESA's billion star surveyor.
Located at the Lagrange point L2, 1.5 million km away from Earth in the opposite direction from the Sun, Gaia scans the entire sky about every three months to map the positions and motions of a billion stars in our Galaxy. In the process, the satellite also picks up objects much closer to home, such as asteroids and comets in the solar system, gathering data that will be used to determine their orbits to unprecedented accuracy.
Gaia is optimised to detect stars, which appear as point sources in its camera, and measure their properties, but it does not routinely return images of celestial objects. To acquire an image of a particular object, a special trick can be used. This is what Gaia astronomers did to ensure that, when the satellite scanned the patch of the sky including Rosetta's comet, the star-mapper camera would capture an image of this iconic object.
“Comet 67P/C-G had just passed its perihelion on 13 August, and calculations predicted that the patch of the sky containing the comet and Rosetta would be scanned by Gaia on 14 September,” comments Fred Jansen, Gaia mission manager and former Rosetta mission manager.
“It was a remarkable occasion: Gaia and Rosetta, two ESA science missions separated by over 260 million kilometres, one looking at the other and its object of study.”
For this special occasion, the astronomers made sure that Gaia’s star-mapper camera would cover that patch of the sky using a special mode in which a full image is recorded and transmitted to the ground instead of point sources only, as in Gaia's normal science mode.
“This symbolic image has a special value for me: it's my present looking at my past,” adds Jansen.
Pictured in the image are the comet's coma and tail, while the nucleus and Rosetta, which was some 300 km from the surface at the time, are both hidden in the innermost pixel. A number of background stars are also sprinkled around the image, which measures about 4.5 arc minutes across.
The image scale, 0.35 arc seconds per pixel, is roughly similar to that of an image of a ground-based image of 67P/C-G that was recently obtained with the 2-m Liverpool Telescope at La Palma, in the Canary Islands.
Besides the special observing mode that was used to obtain the image, the comet was also caught by the on-board detection software as a 'suspected moving object'. Over the three-second observation time, it appeared to move by some 100 km with respect to the background stars, as seen from a distance of about 260 million kilometres.
Over its five-year long mission, Gaia will observe hundreds of comets and repeatedly measure their position to unprecedented accuracy. These data will allow scientists to improve the orbit determination of many comets well beyond the precision that can be achieved with ground-based observations alone; in addition, they will also use Gaia's photometric observations of these comets to investigate their composition and surface properties.
“This is the latest in a succession of Rosetta combinations with data from other ESA missions, including Cluster, Mars Express and also the Earth Observation satellite ENVISAT,” says Matt Taylor, ESA Rosetta project scientist.
.
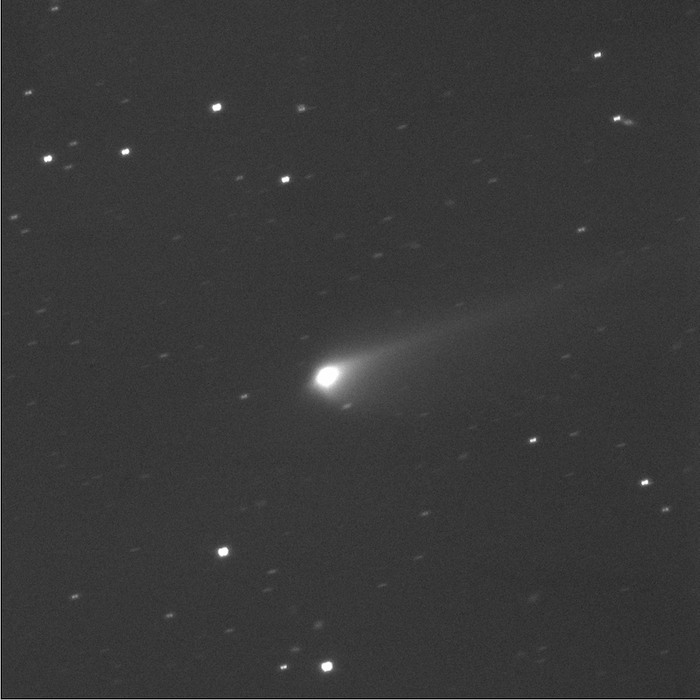
Comet 67P/C-G captured by the 2 m Liverpool Telescope at around 05:30 UT on 8 October 2015. Credit: Colin Snodgrass / Liverpool Telescope
Quelle: ESA
-
Update: 4.11.2015
.
Historische Rosetta-Mission wird mit Absturz in Kometen beendet
Historic Rosetta mission to end with crash into comet
There were other options, but super close-up shots on descent will provide science bonanza.
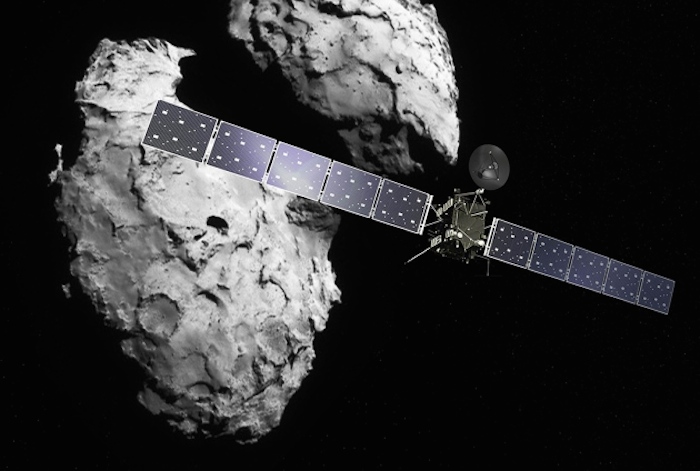
Artist’s impression of the Rosetta orbiter approaching the comet 67P/Churyumov-Gerasimenko.
-
A year since a probe called Philae made history by touching down on a comet, the team that pulled off the feat is plotting a different kind of landing. Next September, the European Space Agency will crash Philae’s mothership Rosetta into the icy dust ball, but as gently as possible.
The dramatic act will bring the mission to an abrupt end — and give Rosetta’s wealth of sensors and instruments their closest view of the comet yet. “The crash landing gives us the best scientific end-of-mission that we can hope for,” says Rosetta project scientist Matt Taylor.
The collision will be emotional for the scientists, some of whom have worked on the mission since its inception in 1993. “There will be a lot of tears,” says Taylor.
Launched in 2004, the Rosetta orbiter caught up with the comet 67P/Churyumov-Gerasimenko ten years later as the rock was travelling from deep in space towards the Sun — and dropped Philae onto the surface a few months later, on 12 November. Scientists have not heard from Philae since July, and don’t know if they will do so again, but Rosetta’s operations to survey the comet from orbit are in full swing. However, the orbiter can’t keep up this work indefinitely. Funding for the mission runs out in September 2016 — and by that time 67P/Churyumov-Gerasimenko will be well on its way back out into deep space, where the solar-powered orbiter will receive too little sunlight to function.
Discussions about what to do with Rosetta when that happens have continued for more than a year. Rosetta flight director Andrea Accomazzo says that, ideally, Rosetta would hibernate while the comet remains in deep space, then be resurrected when 67P again approaches the Sun in 4 or 5 years’ time. But the cold of deep space would probably damage the craft, Accomazzo says; others fear that fuel and other resources would run out. Moreover, many of the mission’s principal investigators (PIs) began their work more than 20 years ago and “there’s no point putting an old experiment with old PIs into hibernation”, jokes Kathrin Altwegg, a planetary scientist at the University of Bern.
Crash-landing Rosetta emerged as the preferred option last year, but only now are orbiter navigators and operators working out how to go about it. Rosetta’s closest encounter with the comet so far was from 8 kilometres above the surface, when it dispatched Philae. The current thinking sees Rosetta spiral down to a similar distance next August before creeping ever closer in elliptical orbits and crashing in September, says mission manager Patrick Martin — but that could still change.
Although Philae sent back some data during its descent, Rosetta has more powerful — and more varied — sensors and instruments. The orbiter will also descend much more slowly than Philae did, allowing it to gather more data and better pictures. Once it gets to 4 kilometres, for example, Rosetta should be able to distinguish between the gases emerging from each of the duck-shaped comet’s two lobes to determine whether the regions vary in composition, says Altwegg, who leads the team behind ROSINA (the Rosetta Orbiter Spectrometer for Ion and Neutral Analysis). That could shed light on the environments in which each was formed.
Rosetta’s cameras will get their best-resolution shots of the comet’s surface yet — less than 1 centimetre per pixel once the craft is within 500 metres of the surface, adds Holger Sierks, PI for Rosetta’s OSIRIS (Optical, Spectroscopic, and Infrared Remote Imaging System). This will allow researchers to look at surface properties and link these to comet activity that Rosetta has observed from orbit.
Over and out
How far into the descent Rosetta will be able to send data back to mission control will depend on whether engineers can design the final trajectory such that the craft crashes on the side of the comet that faces Earth. Navigating while close to the comet will be difficult because the body’s gravitational field is uneven, but spacecraft-operations manager Sylvain Lodiot hopes that the orbiter will transmit until the very end.
The crash will definitely be a hard stop to the mission, he says, however gentle the landing. Designed to manoeuvre in orbit, once Rosetta is on the comet’s surface it will no longer be able to point its antenna to communicate with Earth. Similarly, it will not be able to angle its solar array, so it will lose power, says Lodiot. “Once we touch, hit or crash, whatever you want to call it, it’s game over.”
Before then, though, the mission still has much to accomplish. As the comet approached the Sun, it heated up, with vaporizing ice causing more and more gas and dust to stream from its surface. Rosetta had to retreat into a wider orbit to stop the dust from confusing its navigation system. But now that the comet is speeding away from the Sun, mission scientists are relishing the opportunity to steer Rosetta back in. Priorities will then be to get images that would enable comparisons of the comet before and after its swing around the Sun, as well as a close-up of the southern hemisphere, which was largely in darkness until May and will disappear back out of view in March.
Rosetta will also resume listening out for Philae. Given the huge public interest in anything to do with the lander, Rosetta’s finale will make for a fitting end to the story, adds Altwegg. “This way Rosetta gets to live happily ever after on the comet with Philae.”
Quelle: nature
-
Update: 10.11.2015
.
A FALL OF COMET DUST AND A FIELD OF BOULDERS
From the earliest clear views of comet 67P/Churyumov-Gerasimenko it was obvious that the surface is a collection of contrasts: smooth plains, imposing craggy cliffs and scatterings of boulders. Rosetta scientists are now digging into the detail to explain how some of these features may have arisen and what this means for our understanding of comets.
.
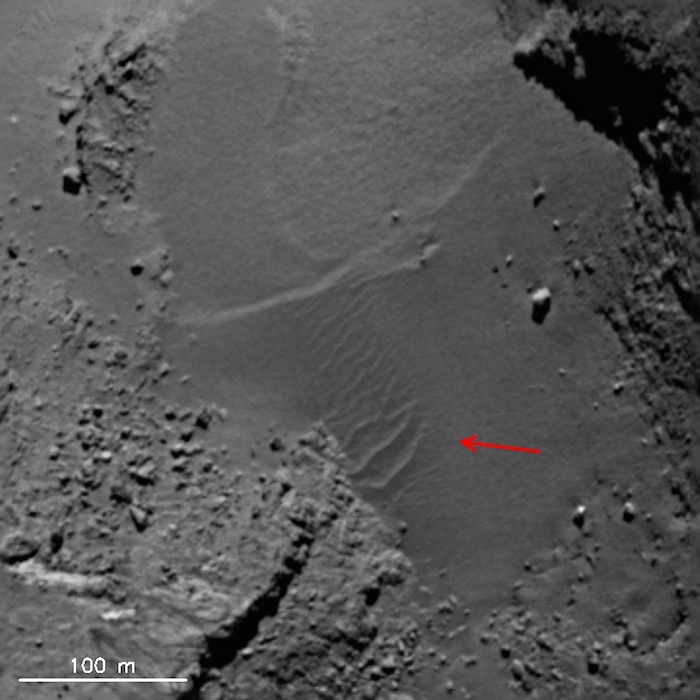
Ripples in the Hapi (neck) region are attributed to a phenomenon known as airfall.
Image credit: see below.
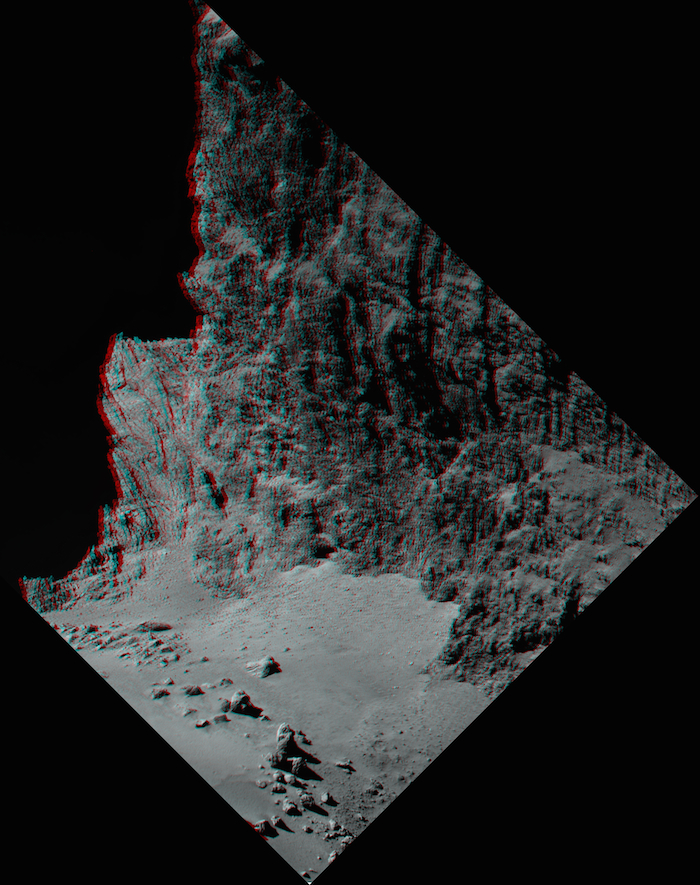
Boulder field in Hapi region. (Individual frames used for the anaglyph can be found below.) Image credit: see below.
-
The study of comet 67P/C-G reveals a dramatic surface environment where considerable amounts of material – up to 1000 kg per second – are ejected from the comet. Not all of this makes it into space, instead some falls back to coat the nucleus.
These small, solid particles – typically with sizes ranging from micrometres to tens of centimetres – are ejected when icy material sublimes. As the ice turns from solid to gas, it escapes into space, propelling the solid particles with it. The smallest of these expelled dust grains – millimeter-sized or smaller – obtain sufficient velocity to escape the influence of the comet and become part of the comet’s tail, which can stretch for millions of kilometres through space.
But some of the larger particles (centimetre-sized or greater) fall back, meaning that particles from one part of the comet can descend to the surface on another part of the comet’s double-lobed nucleus. This ‘airfall’ creates smooth plains that can be as much as a few metres thick.
Nicolas Thomas from Universität Bern, Switzerland, and collaborators have used data from OSIRIS, the science camera on Rosetta, to study these dusty plains. They then used computer models to investigate the mechanisms at work.
The neck, where the two lobes join together and which has been named the Hapi region, has been notably active and so provided an obvious site for investigation. Computer models show that particles ejected from Hapi with speeds below 0.8 m/s will become airfall.
Within the neck, there are ripples in the dust that resemble wind-blown features on Earth and other planets. To understand how these are produced, the team modeled the gas dynamics near the surface of the comet. They found that escaping gas could quickly reach 500 m/s.
Although this speed would partly compensate for the extremely low gas density around the comet, the escaping gas would need to be located very close to the dust deposits to allow the ripples to form. This led the team to investigate other possibilities.
On Earth, the major force to overcome when sculpting wind-blown ripples is gravity holding the grains in place. On the comet, where gravity is minuscule, the major hurdle is the cohesive forces between the dust grains holding them together. This realisation opened another possible formation scenario.
A second computer model showed that as the airfall hits the surface, it dislodges the blanket of particles already there. This makes them more susceptible to weaker gas forces, and also allows the comet’s feeble gravitational field to pull them into the linear features seen in the images.
Although there are many details that remain to be calculated, it is now clear that the airfall phenomenon is an important process in defining the surface properties of a significant fraction of 67P’s nucleus.
Dusty plains are not the only thing covering the surface of the comet. There are also boulders scattered across the dramatic landscape. Maurizio Pajola, University of Padova, Italy, and colleagues have identified 3546 boulders larger than 7 metres in size.
They studied OSIRIS images taken on 5 and 6 August 2014, when Rosetta was close enough to resolve 2.44-2.03 metres per pixel. On an imaged surface of 36.4 km^2 – this is 75% of the total surface of 67P – the number of boulders larger than 7 metres averages to about 100/km^2. Knowing what the boulder distribution was on most of the comet's surface was a crucial input, provided by Maurizio and by Jean-Baptiste Vincent, to the landing site selection process last year. Since then, Maurizio and his colleagues have been able to study the boulders and their distribution in more detail.
These boulders are not uniformly distributed across the comet. On the smaller lobe, often dubbed the head, there are more smaller boulders than on the larger lobe, called the body. In particular, the size distributions are related to how fractured the formation area is. Thermal stress causes fractures on the surface, which can dislodge blocks - forming new boulders - and there is also a continuous fragmentation of boulders that have already been formed. By examining the frequency with which boulders greater than 7 metres are found on the head, the neck and the body of the comet, the team shows that the head is more fractured than the body: the size-distribution for the head is steeper than that of the body.
In the neck region, there is more uniformity between the number of large and small boulders. One particularly intriguing boulder field (see anaglyph above) occurs here and the team suggests that it was created by blocks falling from the cliffs of Hathor, which are located on the body of the comet.
The team has identified three possible reasons why there are fewer small boulders in this field. It could be firstly, because small icy boulders have sublimed away; secondly, small icy boulders could have been ejected from the comet’s surface by outgassing events; thirdly, airfall could have covered the smallest boulders removing them from view. It could be any one or all three mechanisms at work simultaneously.
In all of this work, planetary scientists are starting to build a picture of the processes at work on 67P. It is clear that the comet is a place of sudden change and gradual evolution, making it all the more fascinating to watch as Rosetta’s mission continues.
Quelle: ESA
4218 Views