.
Captured: antimatter from the centre of the Earth
Physicists are probing the secrets of the planet’s inner workings by capturing elusive antimatter particles. Belinda Smith explains.
The middle layer of our planet is a mystery. It makes up 84% of the Earth’s volume, but we’ve little idea what’s in there or how much heat it produces, or how it affects the plate tectonics our planet depends on. That’s about to change thanks to a technique that measures ghostly anti-particles slipping out of their radioactive graves in the mantle below.
An international team at Italy’s Gran Sasso National Laboratory published the calculations in Physical Review D in August. Their sums suggest that more than half our planet’s internal heat comes from radioactive decay within the mantle.
“We know more about the heavens than what’s happening below”
The findings have been welcomed by researchers eager to plumb the mystery of the mantle. “We know more about the heavens than what’s happening below,” says Zheng-Xiang Li, geoscientist at Curtin University in Perth, Australia.
The crusty outer layer of Earth is well understood, but the mantle below is a mystery. We do know it is 2,900 kilometres thick. But efforts to drill through the crust – which is 40 kilometres thick, on average – to reach the mantle have failed. The furthest we got was 12 kilometres when Russian engineers drilled the Kola Superdeep Borehole, the world’s deepest hole. They drilled for 20 years but as the drills neared the mantle, the intense heat wrecked the machinery. Funding ran out in 2005 and the site has been abandoned since 2008.
So geologists use indirect techniques to make their investigations. The mantle (and crust) contains radioactive uranium and thorium. As they decay into lighter elements, they emit ghostly geoneutrinos that pass through rock as if it wasn’t there.
You might already be familiar with a closely related particle, neutrinos. These are uncharged, inert, ghostly particles produced by fusion reactions in the Sun, distant supernovae and other cosmic events. Because they rarely interact with other particles, they’re exceptionally hard to detect.
Geoneutrinos are their antimatter equivalent – and are equally elusive. (Antimatter is composed of antiparticles. They have the same mass as particles of ordinary matter but an opposite charge.)
When a neutron inside a thorium or uranium atom decays, a proton, an electron and a geoneutrino are emitted. While protons and electrons are everywhere, the comparitively rare geoneutrino is the perfect marker for measuring remote radioactivity.
To pick up geoneutrinos’ infrequent interactions, detectors must be big and well shielded from stray, more interactive particles. Cue the Borexino instrument at the Gran Sasso National Laboratory in Italy. “It’s the only tool we have to study the interior of the Earth,” says Aldo Ianni, a physicist at Canfranc Laboratory in Spain as well as a member of the Borexino team.
.
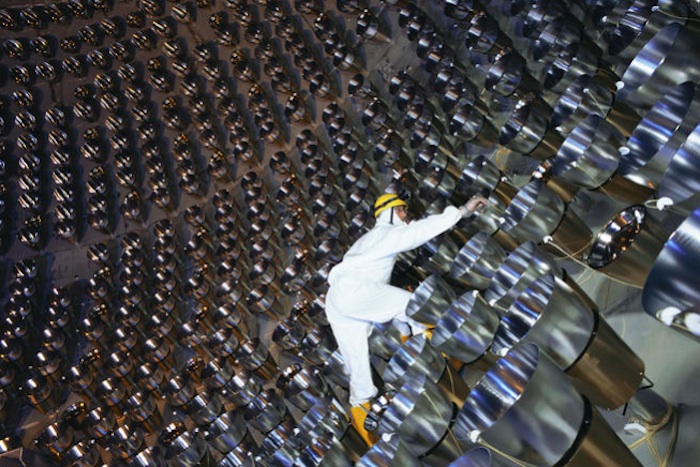
A scientist checks the photodetectors inside the Borexino neutrino detector at Gran Sasso Laboratories.
CREDIT: VOLKER STEGER/GETTYIMAGES
-
It consists of an 18-metre steel box buried 800 metres underground. When a geoneutrino slips past the layers of steel and pure water designed to keep other particles out, it eventually reaches a central tank filled with a hydrogen-rich fluid.
In this environment, if a geoneutrino crashes into a proton, they produce a positron (an electron with a positive charge) and a neutron. The positron immediately annihilates and as it does, it puffs out a burst of light picked up by super-sensitive photodetectors placed around the tank.
The neutron, on the other hand, is captured by a hydrogen atom, which becomes the slightly heavier deuterium. As it slots the extra neutron in its nucleus it shoots out a gamma ray – which is also spotted by photodetectors.
The time delay between the annihilating positron’s flash and the gamma ray is a reliable 250 milliseconds, “a very strong signature” that a geoneutrino has been captured, Ianni says.
Ianni’s team picked up 77 flashes with the geoneutrino signature from December 2007 to March 2015. Some 53 were traced to nearby nuclear reactors (a known geoneutrino source). That left 24. Crust radioactivity accounted for 12 flashes leaving 12 unaccounted for. The researchers believe there’s a 98% chance at least some of these came from the mantle.
Based on the energies of the mantle geoneutrinos they spotted, the team could calculate that 50-70% of the heat the Earth emits into space is generated by radioactivity in the mantle – up to double previous estimates.
Knowing how much heat the mantle produces, geophysicists may one day be able to map how heat is transferred from Earth’s hot liquid core via the mantle to the crust. And because motion in the mantle tugs on the overlying crust, mapping this flow could give new insights into the processes driving plate tectonics and earthquakes, volcanic eruptions and lava flows, says Li.
The Borexino researchers plan to run the detector for another three to five years, until the light-sensitive photodetectors age.
There’s more data to come though, both from Borexino and the KamLAND antineutrino detector, buried in an old mine shaft under the Japanese Alps. And a new detector – the SNO+ underground experiment in Ontario, Canada – is under construction. The more the better, Ianni says, to “combine data and disentangle the models, and build a bridge between particle physics and geophysics.”
Quelle: Cosmos
4282 Views