.
Discoveries of extreme life here on Earth often provoke speculation about what might lurk in other worlds. And so it was, when I reported on January 21 that fish were found living in an isolated corner of the ocean beneath 740 meters of ice in Antarctica: People asked what this might mean for finding life on distant worlds such as Europa, a moon of Jupiter that very likely harbors an ocean of liquid water beneath a crust of ice.
Astrobiologists wax poetic about the possibility that we might someday find carpets of microbial slime clinging to the aquatic underside of Europa’s ice, but might they be setting their sights too low? Might there be something more exciting gliding through Europa’s waters, like the spidery-legged, bioluminescent xeno-arachnids envisioned in a sci-fi sketch recently published in Nature? “The question will always be energy,” says Britney Schmidt, a planetary scientist at Georgia Institute of Technology who studies Europa as a possible habitat of life. “Fish require a lot of energy—a lot more than microbes.” (Scientific American is part of Nature Publishing Group.)
Fish require a multilevel ecosystem—the bioenergetic equivalent of a financial pyramid scheme. At the bottom of an aquatic ecosystem are single-celled microbes that use energy from either sunlight or chemical sources to pluck molecules of carbon dioxide out of the water in order to grow. Microscopic creatures called protists eat the microbes. And in the newly discovered Antarctic ecosystem crustaceans may eat the protists, and fish, at the top of the pyramid, may eat the crustaceans.
This transfer of carbon—or energy—up the food web is inefficient, says John Priscu, a microbial ecologist from Montana State University who was part of the team that discovered the Antarctic fish this month. “You lose about 90 percent of the energy as you go [each step] up a food web,” he says. So for every kilogram of fish living in that ecosystem under the ice, you might need up to 1,000 kilograms of microbes at the base of the food web to support it. Even if the newly discovered fish turn out to feed on microbes directly, that still means at least 10 kilograms of microbes per kilogram of fish.
It is this constraint—the extravagant energetic needs of animals, and the limited supply of energy—that will probably determine whether a food web with complex life could exist on Europa.
Most ecosystems on Earth are powered from the base up by sunlight, which drives carbon fixation through photosynthesis. But any life in Europa’s ocean, under 10 or 20 kilometers of ice, would have to use another source of energy. Studies of lakes sealed beneath hundreds of meters of ice in Antarctica have provided a compelling picture of how this might work.
The same team that discovered the ocean fish in Antarctica this year also drilled into one of these subglacial lakes in January 2013: Lake Whillans, which sits under 800 meters of ice, roughly 100 kilometers inland from where the fish were discovered—both expeditions were supported by the National Science Foundation (NSF). They found that Lake Whillans was thriving, with roughly 130,000 microbial cells per milliliter of water. But far more interesting was just how non-hostile this supposedly “extreme” subglacial environment turned out to be. At –0.5 degree Celsius, it was slightly warmer than the oceans surrounding Antarctica. And despite having sat under ice for thousands of years, it still contained levels of oxygen that some marine animals, such as brittle stars and worms, can actually survive on.
Ironically, the same shell of ice that cuts Lake Whillans off from the outside world also provides it with a steady supply of oxygen. Ambient geothermal heat emanating up from the seafloor melts the underside of the ice sheet at a rate of several penny thicknesses per year. This liberates ancient air bubbles that were trapped in the ice as it formed from falling snow thousands of years earlier.
Genetic studies suggest that the microbes in Lake Whillans use this oxygen to “burn” ammonium and iron minerals seeping up from the sediments below—providing energy to fix carbon—effectively replacing photosynthesis. “It’s like a battery,” says Priscu, whose laboratory retrieved and analyzed some of the samples from the lake in 2013. “The ice has oxidants and the sediments have reductants, and life evolves to fill the gap—the free energy gap.”
Kevin Hand, an astrobiologist at NASA’s Jet Propulsion Laboratory, believes that this same scheme also exists on Europa. Using spectral readings from telescopes at the Keck Observatory in Hawaii, Hand has found high levels of oxidative chemicals such as sulfate, oxygen, sulfur dioxide and hydrogen peroxide on Europa’s surface, which are produced as ionizing radiation from Jupiter scours it, splitting apart water molecules and sulfur compounds in the uppermost layers of its ice. Living organisms could use these oxidizing chemicals to burn fuels such as iron or methane seeping up from the rocky bottom of Europa’s ocean.
What makes this possible is that Europa seems to be geologically active, allowing these supplies of fuel and oxidants to be transported, mixed and constantly renewed. Schimdt has found evidence that warm ocean currents and convective forces beneath Europa’s frozen shell can cause large blocks of ice to overturn and melt, bringing vast pockets of water, sometimes holding as much liquid as all of the Great Lakes combined, to within several kilometers of the moon’s icy surface.
Scientists analyzing the cracks and ridges on Europa’s surface find that its icy skin is also slowly recycled through a process similar to continental subduction on Earth, with one icy plate slipping and buckling under the edge of another. The plate sinks and eventually melts back into the ocean below, carrying with it the oxidative chemicals that were formed on the surface.
The key to predicting what kind of life Europa’s ocean could support will be figuring out how quickly this occurs and how many thousands or millions of tons of oxidative chemicals are formed on the surface and injected into the ocean each year. The range of uncertainty is huge, with estimates of Europa’s energy supply varying by orders of magnitude. Refining these calculations will go a long way toward determining what kind of life might exist there—microbial slime or feathery-lobed xeno-arachnids.
Schmidt is part of a team of scientists in the early stages of developing a NASA mission, called Europa Clipper, which will investigate these questions by placing a reconnaissance spacecraft in orbit around Jupiter. Clipper will periodically swoop down to as low as 25 to 100 kilometers above Europa’s surface. It will use ice-penetrating radar to measure the thickness of the moon’s ice shell, map its internal rifts and faults (clues to the tempo of its geologic activity) and locate pockets of water near the surface. An onboard magnetometer will measure the depth and saltiness of the ocean and a spectrometer will measure chemicals in Europa’s uppermost layers of ice. Clipper would take a few years to build and launch, assuming it gets funded.
In the meantime other interesting questions linger about the recent discoveries in Antarctica. One such question is why fish were found along the ice-covered beach line of Antarctica, where its glaciers begin to float on the ocean, but only microbes were found 100 kilometers upstream in Lake Whillans. Both environments contain oxygen. Both hover around the same temperature. And both ecosystems will likely turn out to be powered by the same source of energy: microbes oxidizing ammonium, iron, sulfur and possibly methane in order to fix carbon.
Lake Whillans and its sister lakes in that region of Antarctica represent unforgiving environments for multiple reasons. They are shallow and transient—shifting their locations from one decade to the next, Schimdt says, “more like mud puddles.” That instability could make it hard for animals to hang on over tens of thousands of years. If some chance event exterminates them—for example the glaciers freezing fast onto their beds—then the likelihood of new animals making it in from the outside to recolonize the lakes afterward is extremely low.
I traveled with the expedition that drilled and sampled Lake Whillans in 2013, and during that time I frequently asked people what they expected to find in the lake. Perhaps it’s no surprise that everyone expected to find only microbes. But now the discovery of animals in an isolated, ice-covered nook of the ocean seems to have jarred people’s thoughts into a new space.
Priscu is quick to point out that the Lake Whillans microbes were actually fixing carbon about as rapidly as what has been seen in some ice-covered parts of the ocean that are known to harbor fish. “I would be surprised if there are fish [in the lake], but there’s enough energy for them” there, he says. “If we get another grant from NSF, it would be interesting to put down a fish trap and let it sit.”
Quelle: Scientific American
-
Discovery: Fish Live beneath Antarctica
Scientists find translucent fish in a wedge of water hidden under 740 meters of ice, 850 kilometers from sunlight
.
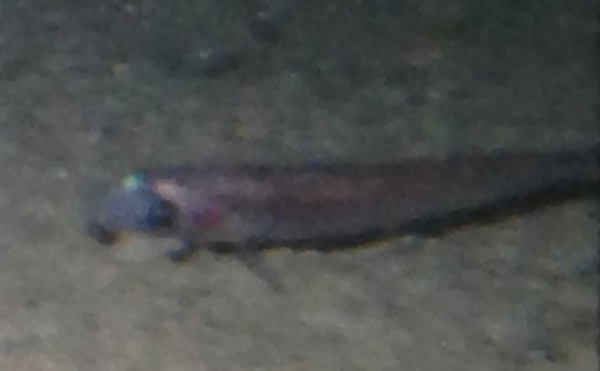
The first low-resolution image of a translucent fish that researchers discovered incredibly far beneath Antarctica’s ice reveals two black eyes and various internal organs (colored blobs). Credit: Whillans Ice Stream Subglacial Access Research Drilling Project
.
Stunned researchers in Antarctica have discovered fish and other aquatic animals living in perpetual darkness and cold, beneath a roof of ice 740 meters thick. The animals inhabit a wedge of seawater only 10 meters deep, sealed between the ice above and a barren, rocky seafloor below—a location so remote and hostile the many scientists expected to find nothing but scant microbial life.
A team of ice drillers and scientists made the discovery after lowering a small, custom-built robot down a narrow hole they bored through the Ross Ice Shelf, a slab of glacial ice the size of France that hangs off the coastline of Antarctica and floats on the ocean. The remote water they tapped sits beneath the back corner of the floating shelf, where the shelf meets what would be the shore of Antarctica if all that ice were removed. The spot sits 850 kilometers from the outer edge of the ice shelf, the nearest place where the ocean is in contact with sunlight that allows tiny plankton to grow and sustain a food chain.
“I’m surprised,” says Ross Powell, a 63-year old glacial geologist from Northern Illinois University who co-led the expedition with two other scientists. Powell spoke with me via satellite phone from the remote location on the West Antarctic Ice Sheet, where 40 scientists, ice drillers and technicians were dropped by ski-mounted planes. “I’ve worked in this area for my whole career,” he says—studying the underbellies where glaciers flow into oceans. “You get the picture of these areas having very little food, being desolate, not supporting much life.” The ecosystem has somehow managed to survive incredibly far from sunlight, the source of energy that drives most life on Earth. The discovery provides insight into what kind of complex but undiscovered life might inhabit the vast areas beneath Antarctica’s ice shelves—comprising more than a million square kilometers of unexplored seafloor.
The expedition, funded by the National Science Foundation, had ventured to this location to investigate the history and long-term stability of the Whillans Ice Stream, a major glacier that flows off the coast of Antarctica and feeds into the Ross Ice Shelf. The expedition began in December as tractors towed massive sleds holding more than 400 metric tons of fuel and equipment to a remote location 630 kilometers from the South Pole and 1,000 kilometers from the nearest permanent base.
In early January the team began an unprecedented effort to drill through the ice to reach a place called the grounding zone—essentially, a subglacial beach where the glacier transitions from resting on bedrock to floating on sea water as it oozes off the edge of the continent. A team of ice drillers from the University of Nebraska-Lincoln (U.N.L.) used a jet of hot water from the end of a Kevlar hose a kilometer long and as big around as an ankle to melt a hole through the ice into the seawater below.
Until now no one had ever directly observed the grounding zone of a major Antarctic glacier. And from the moment the hole was first opened on January 7 Pacific time, it appeared that this place didn’t hold much in the way of life.
Deceived by lifeless mud
A downward-facing video camera lowered through the borehole found a barren sea bottom—“rocky, like a lunar surface,” Powell says. Even deep “abyssal” ocean floors three or four kilometers deep in the ocean usually show some signs of animal life: the tracks of crustaceans that have scuttled over the mud, or piles of mud that worms have ejected from their burrows. But the camera showed nothing of the sort. Cores of mud that the team gently plucked from the bottom also showed no signs that anything had ever burrowed through underneath. And seawater lifted from the bottom in bottles was found to be crystal clear—suggesting that the water was only sparsely populated with microbes, and certainly not enough of them for animals to graze and sustain themselves on.
“The water’s so clear—there’s just not much food,” says Trista Vick-Majors, on a separate satellite call. Vick-Majors is a PhD microbiology student from Montana State University, who handled samples of water lifted from the bottom. What’s more, sediments in the sea floor were packed with quartz, a mineral that holds little nutritional value for microbes. When mud is raised from the bottom of an ocean or lake, it is often possible to smell gases such as hydrogen sulfide that are produced by microbes—“your nose is a great detector of microbial activity,” says Alex Michaud, a microbiology PhD student also from Montana State who is working with the sediment samples. “But I don’t smell anything.”
The revelation that something larger lived down there in the dark came eight days after the hole was opened, on January 15 Pacific time.
The finding depended on a skinny, 1.5 meter-long robot called Deep-SCINI, with eyes made of reinforced, pressure-resistant sapphire crystal and a streamlined body of aluminum rods and high-tech, “syntactic” foam comprising millions of tiny, hollow glass beads.
Deep-SCINI, a remotely operated vehicle (ROV), is designed to slip down a narrow, icy borehole and explore the water cavity below. It carries sapphire-shielded cameras, a grabber arm, water-samplers and other instruments. Robert Zook and Justin Burnett, from the U.N.L. ice-drilling program, had worked day and night to finish building it in time for the expedition, flying to New Zealand and then Antarctica with it in their carry-on cases.
Just after lunch on January 16 workers in hard hats coupled Deep-SCINI to a fiber optic cable as thick as a garden hose. A winch atop the drill platform hummed into action, unwinding cable from a giant spool, lowering the ROV down the hole. Deep-SCINI had “flown” (as Zook called it) in swimming pools and tested once in a pressure chamber to confirm that it could survive the deep ocean. But this would be its first real dive, deeper down through glacial ice than any ROV had ever ventured.
Fish!
A dozen people crowded inside a compact control room, built inside a cargo container mounted on skis, to watch the ROV’s maiden flight play out on several video monitors.
The view down the hole was obscured by a block of concrete hanging from Deep-SCINI’s claw—intended to keep the craft vertical in the narrow borehole, only three quarters of a meter across. Instead, for 45 minutes as the ROV crept downward, its side-looking camera caught images of dark debris layers on the walls of the hole, trapped deep in the ice, possibly the remains of volcanic ash or other dust deposited on the ice surface thousands of years ago. The researchers discovered the layers several days earlier when they first drilled the hole. They later found pebbles at the bottom, suggesting that the underside of the ice sheet might be melting faster than people had thought (see my story on that discovery here). Fast melting could allow the massive glacier on land to slide into the sea more quickly that scientists had anticipated.
Finally the walls of the hole, lit by Deep-SCINI’s lamp, fell away into darkness. The ROV emerged into a boundless void of pitch-black water beneath the ice. Bright flecks streamed down like falling stars past the side-looking camera—the light of Deep-SCINI’s lamps reflecting off bits of sand, trapped in the ice for thousands of years, now falling to the seafloor somewhere below after being disturbed by the robot’s descent.
The ROV reached the rocky bottom. Burnett (a PhD student), sitting at the controls in the cargo container, nudged a lever: the claw opened, the concrete weight came to rest on the bottom and Deep-SCINI righted to a horizontal position. Zook, the self-taught engineer who conceived this ROV and designed much of it, sat beside Burnett, operating cameras and displays. People standing in the unlit room stared into the blackness of the video monitors. Here and there they glimpsed hints of motion just past the reach of the lights: a bit of falling debris that suddenly changed direction, or a shadow flitting through a corner.
Burnett and Zook continually worked around problems as they piloted an ROV clearly still in its test stage. An overheating problem—ironic, in this place—forced them to operate the thrusters below capacity. No navigation system had yet been built into the ROV, so they maneuvered using tricks—flying from one large rock on the bottom to another, or having the winch operator reel in a couple meters of cable, to tug the ROV from behind and point it away from the hole. They found themselves working on an unexpectedly short leash—forced to stay within 20 or 30 meters of the hole by a tether cable snagged somewhere above.
At last Burnett and Zook brought Deep-SCINI to a standstill a meter above the bottom, while they adjusted their controls. People in the cargo container stared at an image of the sea floor panned out on one of the video monitors, captured by the forward-looking camera. Then someone started to yell and point. All eyes swung to the screen with the down-looking camera.
A graceful, undulating shadow glided across its view, tapered front to back like an exclamation point—the shadow cast by a bulb-eyed fish. Then people saw the creature casting that shadow: bluish-brownish-pinkish, as long as a butter knife, its internal organs showing through its translucent body.
The room erupted into cheering, clapping and gasps. “It was just amazing,” recalls Powell.
Bored out of their minds
Deep-SCINI stayed down in the wedge of seawater for six hours. When Burnett parked it on the bottom, a fish—watching, sitting motionless far off across the bottom, gradually came closer, swimming from one motionless perch to another over a period of 20 minutes until it came within half an arm length of the camera. These fish, attracted perhaps by the novelty of light, were “curious and docile,” Zook says. “I think they’re bored. I know I would be.”
All told, the ROV encountered 20 or 30 fishes that day. “It was clear they were a community living there,” Powell says, “not just a chance encounter.” The translucent fish were the largest. But Deep-SCINI also encountered two other types of smaller fish—one blackish, another orange—plus dozens of red, shrimpy crustaceans flitting about, as well as a handful of other marine invertebrates that the team has so far declined to describe.
To the microbiologists who were present, the most exciting thing was not the discovery of fish itself, but rather what it says about this remote, unexplored environment. Just three days before the discovery, Brent Christner, a microbiologist from Louisiana State University (L.S.U.) with years of experience studying ice-covered Antarctic lakes, had agreed with Vick-Majors that life in the water would be limited to microbes with sluggish metabolic rates. “We have to ask what they’re eating,” he says, when I asked later on about the fishes. “Food is in short supply and any energy gained is hard-won. This is a tough place to live.”
One source of food could be small plankton, grown in the sunlit waters of the Ross Sea then swept by currents under the ice shelf. But oceanographic models suggest that this food would have to drift six or seven years under the dark of the ice shelf before reaching the Whillans grounding zone, encountering plenty of other by other animals along the way. “The water will be pretty chewed on by the time it gets here,” Vick-Majors says.
The ecosystem could also be powered by chemical energy derived from Earth's interior, rather than sunlight. Bacteria and other microbes might feed on mineral grains dropped from the underside of the ice or flushed into the sea water by subglacial rivers flowing out from beneath the West Antarctic Ice Sheet. The microbes at the bottom of the food chain could also be fed by ammonium or methane seeping up from ancient marine sediments hundreds of meters below. In fact, two years ago when this same team drilled into a subglacial lake 100 kilometers upstream, they found an ecosystem that fueled itself largely on ammonium—although in that case, the ecosystem included only microbes, with no animals present.
People had speculated that the nutrient-poor environment beneath Antarctica’s large ice shelves would resemble another underfed habitat—the world’s vast, abyssal sea floors sitting below 3,000 meters. But important differences are already emerging: The muddy floors of the oceanic abyss are populated by worms and other animals that feed on bits of rotting detritus that rain down from above. But the mud cores brought up so far from the Whillans grounding zone haven’t revealed such animals. Nor did Deep-SCINI’s cameras. “We saw no established epi-benthic community,” Powell says. “Everything living there can move.”
These new results are still extremely preliminary but a similar pattern was seen in the late 1970s when a hole was briefly melted through another part of the Ross Ice Shelf not as far inland—the so-called J9 borehole, which reached a layer of sea water 240 meters thick, sitting 430 kilometers in from the edge of the ice. Fish and crustaceans were seen in the water, but nothing was spotted in the mud. The lack of mud dwellers might indicate that animals living this far under the ice shelf must be mobile enough to follow intermittent food sources from place to place.
Whatever the ultimate energy source, bacteria would serve as food for microscopic organisms called protists, crustaceans would eat the protists and fishes would eat the crustaceans—or sometimes, one another’s young—says Arthur DeVries, a biologist at the University of Illinois at Urbana-Champaign. DeVries was not on this expedition but has spent 50 years studying fishes living near the exposed front of the Ross Ice Shelf.
Whether the fishes themselves represent something truly novel to science remains to be seen. Photographs and videos will have to be extensively analyzed and the results published in a peer-reviewed journal before the team is likely to say much more. The fishes could turn out to belong to a single family, called the Nototheniidae, DeVries says. These fishes began to dominate Antarctica starting around 35 million years ago, when the continent and its surrounding oceans began to cool precipitously, and the fishes evolved proteins that helped them avoid freezing solid.
Years of data to come
Even with the joyful discovery of fishes, the day was far from over. Back in the control room Burnett and Zook struggled to overcome technical difficulties and bring Deep-SCINI back to the surface. A buoyant string, floating like a helium balloon above the concrete block that it was tied to, helped them find their way back to the block—and hence to the borehole that would be the robot’s exit. Even then, the duo had to grab hold of the weight again, in order to put the ROV back in its vertical pose and ascend the hole. One of Deep-SCINI’s cameras had been bumped out of position during the dive, so it no longer focused on the claw. The two operators spent 45 minutes trying to snag it before finally succeeding.
“We had a minor miracle,” says Zook, of Deep-SCINI’s maiden flight. Antarctica’s harsh conditions tend to punish innovation, he notes: “The rule of thumb down here is that any new technological thing does not work for the first deployment.”
Two hours after Deep-SCINI was hoisted into daylight atop the drill platform, another instrument was lowered and parked at the bottom for 20 hours to measure gases, currents, temperatures and salinity– all expected to change as remote ocean tides push and pull at this deep recess of water. Throughout that time, a down-looking lamp and camera repeatedly attracted visitors—reddish crustaceans or inquisitive fishes.
Up top, Zook fashioned some window screening into a trap for crustaceans. Michaud built a fish trap using parts from a lobster trap that Zook had purchased as a joke at a sporting goods store in New Zealand while in route to Antarctica. They have so far caught a handful of crustaceans for further scientific study—but no fishes, at this writing.
Even as all of this went on, work continued for an expedition whose overall goal was to understand the behavior of the glacier as it meets the ocean. Slawek Tulaczyk, a glaciologist from the University of California, Santa Cruz, who co-led the expedition with Powell and another scientist, missed the fish hubbub because he was a short distance away on the ice surface, lowering a string of sensors into another hole that had just been melted through the ice. The hole will refreeze, sealing the string in the ice shelf. For years to come it will record temperatures up and down the ice, and also in the water below. It will record the ebb and flow of tides, and pulses of cloudy water from subglacial rivers flowing into the ocean. Tilt meters will measure how the ice shelf flexes in response to the tide that rises and falls a meter beneath it each day. Seismic sensors will record the pops and snaps as crevasses erupt on the underside of the flexing ice. The goal is to find out how much heat and mechanical stress is being delivered to the grounding zone of the Whillans Ice Stream.
“I know it sounds wonky,” Tulaczyk wrote via email—by which he might have meant, less cute and charismatic than bug-eyed fishes. But this data, he says, will fill in some key unknowns about how quickly ice will melt from the underbelly of this glacier.
Right now the Whillans Ice Stream is actually slowing down a little each year—a rarity among glaciers in Antarctica—part of a complex cycle of intermittent stops and starts that occur over hundreds of years in several glaciers that feed into this part of the Ross Ice Shelf. Knowing the melt rate at the Whillans grounding zone could shed light on the meaning of last week’s discovery of stones raining down from the underside of the ice there. It could determine whether changes are already underway that could overcome the Whillans’s current slowdown and cause it to accelerate its flow into the ocean once more. All of this is important for understanding how glaciers in this part of Antarctica might contribute to global sea level rise.
Even as the downward camera recorded the comings and goings of fishes for 20 hours on January 15 and 16 Pacific time, Tulaczyk was focused on something else, far more subtle, in the camera’s view. A weight planted on the bottom below the hole was sliding past the camera – slowly at first, then faster. The weight was stationary but the glacier above it had begun to slide: The Whillans Ice Stream is known for its bizarre habit of staying still most of the time but lurching forward twice per day—but this was the best measurement that had ever been obtained.
Those layers of dust or ash that Deep-SCINI documented on its way down the hole will also keep ice guys like Tulaczyk and Powell busy for some time. “It was a great trip down, even before the fish,” says Powell. “It will be a great data set.”
As the microbiologists head home with their water and mud samples they will face the unexpected task of figuring out whether this entire ecosystem, including the fishes, really does sustain itself on methane, ammonium or some other form of chemical energy. “That would be really exciting,” L.S.U.'s Christner says. “Our samples can help answer that.”
Quelle: Scientific American
3941 Views