On Wednesday, Nov. 6, 2024, NASA’s Parker Solar Probe will complete its final Venus gravity assist maneuver, passing within 233 miles (376 km) of Venus’ surface. The flyby will adjust Parker’s trajectory into its final orbital configuration, bringing the spacecraft to within an unprecedented 3.86 million miles of the solar surface on Dec. 24, 2024. It will be the closest any human made object has been to the Sun.
31.12.2023
Nasa mission lines up to 'touch the Sun'
It promises to be a remarkable moment in the history of space exploration.
A year from now, on 24 December, Nasa's Parker Solar Probe will race past the Sun at the astonishing speed of 195 km/s, or 435,000 mph.
No human-made object will have moved so fast nor, indeed, got so close to our star - just 6.1 million km, or 3.8 million miles from the Sun's "surface".
"We are basically almost landing on a star," said Parker project scientist Dr Nour Raouafi.
"This will be a monumental achievement for all humanity. This is equivalent to the Moon landing of 1969," the Johns Hopkins University Applied Physics Laboratory scientist told BBC News.
Parker's speed will come from the immense gravitational pull it feels as it falls towards the Sun. It will be akin to flying from New York to London in under 30 seconds.
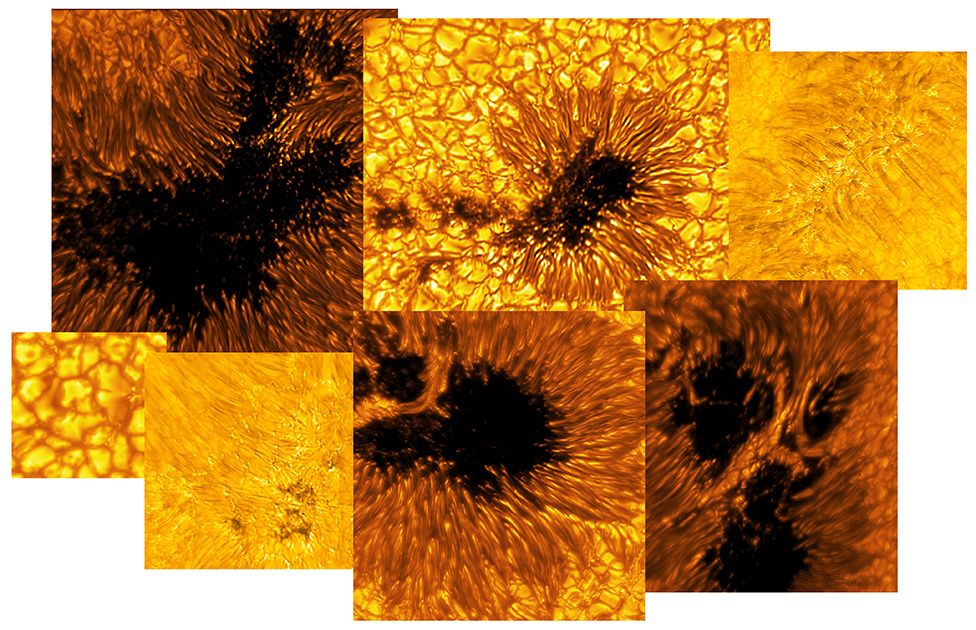
The US space agency's Parker Solar Probe is one of the most audacious missions ever conceived.
Launched in 2018, it has the goal of making repeated, and ever closer, passes of the Sun.
The late 2024 manoeuvre will take Parker to just 4% of the Sun-Earth distance (149 million km/93 million miles).
The challenge Parker faces in doing this will be huge. At perihelion, the point in the probe's orbit nearest the the star, the temperature on the front of the spacecraft will probably reach 1,400C.
Parker's strategy is to get in quick and get out quick, making measurements of the solar environment with a suite of instruments deployed from behind a thick heat shield.
The reward, researchers hope, will be breakthrough knowledge on some key solar processes.
Chief among these is a clearer explanation of the workings of the corona, the Sun's outer atmosphere.
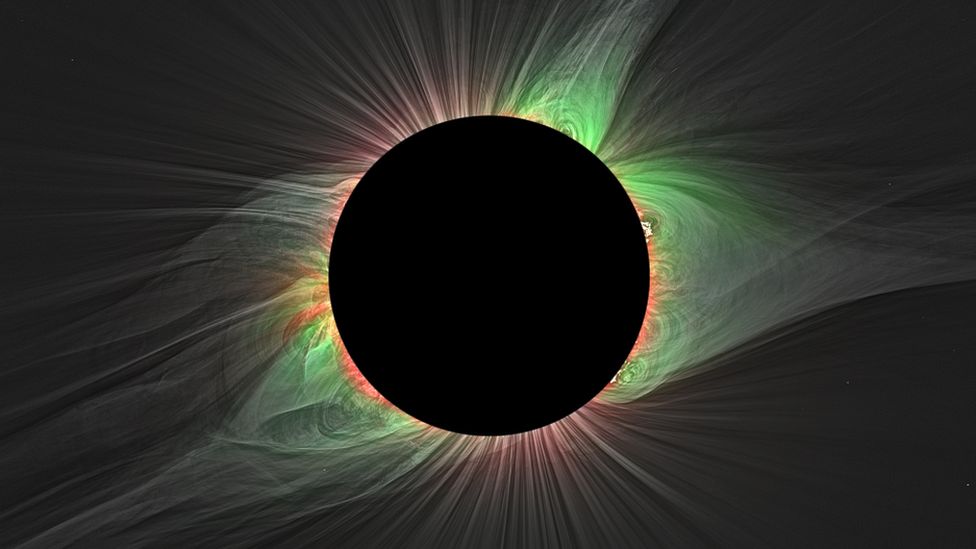
It experiences what seems to be counter-intuitive superheating. The temperature of the Sun at its photosphere, the surface, is roughly 6,000C but within the corona it can reach a staggering million degrees and more.
You'd think temperature would decrease with distance from the star's nuclear core.
It's also within the corona region that the outward flow of charged particles - electrons, protons and heavy ions - suddenly gets accelerated into a supersonic wind moving at 400 km/s, or 1,000,000 mph.
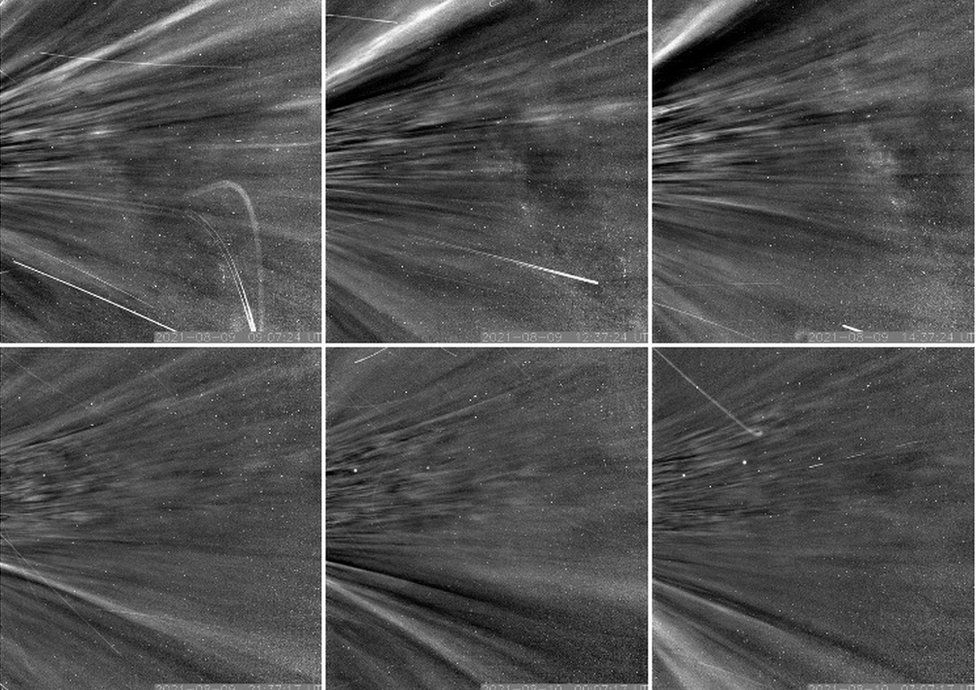
Scientists still can't fully explain this either. But it's critical to improving forecasts of solar behaviour and the phenomenon of "space weather".
The latter refers to the powerful eruptions of particles and magnetic fields from the Sun that can degrade communications on Earth and even knock over power grids. The radiation also poses health risks to astronauts.
"This takes on a new dimension, especially now that we're thinking of sending women and men back to the Moon and even setting up a permanent presence on the lunar surface," Dr Raouafi said.
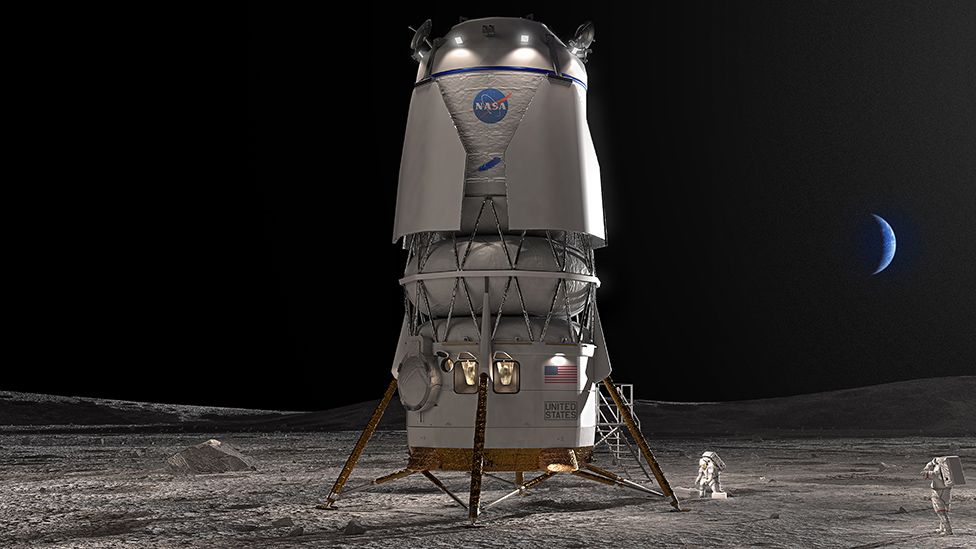
Parker made one of its close approaches to the Sun on Friday. It has three more planned in 2024 before it then swings around Venus on 6 November to help bend its orbit and make 24 December a historic occasion.
The head of science at Nasa is Dr Nicky Fox. She was the lead scientist on Parker before taking up her current role.
She said the major plus of the 24 December flyby would be the length of time the probe got to sit in the corona, far longer than on any previous pass.
"We don't know what we'll find, but we'll be looking for waves in the solar wind associated with the heating," she told BBC News.
"I suspect we'll sense lots of different types of waves which would point to a mix of processes that people have been arguing over for years."
- Listen to Dr Nicky Fox edit the BBC's Today Programme.
The coming year will be the apex of Parker's mission; it won't be able to get any closer to the Sun beyond December, not least because its orbit will no longer afford swingbys of Venus to train its trajectory onto an even tighter path.
But to go any closer would also risk shortening the shadow cast by Parker's big shield, exposing the rear of the spacecraft to intolerable temperatures.
How a NASA Probe Solved a Scorching Solar Mystery
The outer layers of the sun’s atmosphere are a blistering million degrees hotter than its surface. The hidden culprit? Magnetic activity.
Our sun is the best-observed star in the entire universe.
We see its light every day. For centuries, scientists have tracked the dark spots dappling its radiant face, while in recent decades, telescopes in space and on Earth have scrutinized sunbeams in wavelengths spanning the electromagnetic spectrum. Experiments have also sniffed the sun’s atmosphere, captured puffs of the solar wind, collected solar neutrinos and high-energy particles, and mapped our star’s magnetic field — or tried to, since we have yet to really observe the polar regions that are key to learning about the sun’s inner magnetic structure.
For all that scrutiny, however, one crucial question remained embarrassingly unsolved. At its surface, the sun is a toasty 6,000 degrees Celsius. But the outer layers of its atmosphere, called the corona, can be a blistering — and perplexing — 1 million degrees hotter.
You can see that searing sheath of gas during a total solar eclipse, as happened on April 8 above a swath of North America. If you were in the path of totality, you could see the corona as a glowing halo around the moon-shadowed sun.
This year, that halo looked different than the one that appeared during the last North American eclipse, in 2017. Not only is the sun more active now, but you were looking at a structure that we — the scientists who study our home star — have finally come to understand. Observing the sun from afar wasn’t good enough for us to grasp what heats the corona. To solve this and other mysteries, we needed a sun-grazing space probe.
That spacecraft — NASA’s Parker Solar Probe — launched in 2018. As it loops around the sun, dipping in and out of the solar corona, it has collected data that shows us how small-scale magnetic activity within the solar atmosphere makes the solar corona almost inconceivably hot.
From Surface to Sheath
To begin to understand that roasting corona, we need to consider magnetic fields.
The sun’s magnetic engine, called the solar dynamo, lies about 200,000 kilometers beneath the sun’s surface. As it churns, that engine drives solar activity, which waxes and wanes over periods of roughly 11 years. When the sun is more active, solar flares, sunspots and outbursts increase in intensity and frequency (as is happening now, near solar maximum).
At the sun’s surface, magnetic fields accumulate at the boundaries of churning convective cells, known as supergranules, which look like bubbles in a pan of boiling oil on the stove. The constantly boiling solar surface concentrates and strengthens those magnetic fields at the cells’ edges. Those amplified fields then launch transient jets and nanoflares as they interact with solar plasma.
Introduction
Magnetic fields can also erupt through the sun’s surface and produce larger-scale phenomena. In regions where the field is strong, you see dark sunspots and giant magnetic loops. In most places, especially in the lower solar corona and near sunspots, these magnetic arcs are “closed,” with both ends attached to the sun. These closed loops come in various sizes — from minuscule ones to the dramatic, blazing arcsseen during eclipses.
In other places, such loops are torn open. The sun’s searing corona is the source of a supersonic solar wind — streams of charged particles that form a massive protective bubble around the solar system called the heliosphere, which extends far beyond the known planets. These particles carry magnetic fields with them, sometimes all the way into deep space. When that happens, the magnetic loop stretches to the edge of the heliosphere, forming what’s called an “open” magnetic field.
We knew that somehow these magnetic processes must be working together to heat the corona — but how?
Over the years, scientists proposed many explanations for the super hot corona. Some of these treated the solar atmosphere as a fluid, explaining heat transfer as it would occur in a fluid — through messy, turbulent cascades that carry heat from large reservoirs into smaller pockets. Others suggested that magnetic waves originating at the sun’s surface are constantly wiggling and dumping heat into the atmosphere, or that, at the level of particles, some sort of kinetic instability is at work.
In 1988, Eugene Parker, a University of Chicago astrophysicist, arguedthat convection at the solar surface — those churning cells — could tangle magnetic fields that stretched into the corona, thereby building up and storing magnetic energy in the solar atmosphere. When those field lines inevitably snapped and reconnected, he said, the stored magnetic energy would be transferred into the solar atmosphere. There, the energy would heat the atmosphere to high temperatures, leading to nanoflares. (Parker was also responsible for a hypothesis from 1958 suggesting that the superheated corona is the source of the solar wind. Though widely ridiculed at the time, Parker’s idea was correct and foundational to the field of heliophysics.)
Parker’s idea made sense, but we didn’t have sufficient data to verify or falsify any of the explanations, including his. The ways in which we were studying the sun just weren’t up to the challenge.
A New Hope
The turning point came in 2005, when hundreds of solar scientists met in Whistler, British Columbia. I was the meeting’s chair, a role I deliberately assumed in an attempt to integrate the often disjointed approaches of the communities studying the sun and the solar wind.
Until then, the solar community had mostly focused on remote observations of the sun, made by ground-based telescopes, rockets or satellites such as SOHO, a mission led by the European Space Agency (ESA) that had recently been launched and is still operating. The solar-wind community, on the other hand, was busy collecting and analyzing samples of the extended corona using satellites such as NASA’s Advanced Composition Explorer and Ulysses, a joint ESA/NASA mission that flew over the sun’s poles. Our goal for this conference was to merge the often siloed results from these new observatories and see if that might help solve the mystery of the hot corona and how it accelerated the solar wind.

Eugene Parker, seen here in 1977, made predictions about the sun’s magnetic field, corona and solar wind that proved foundational to the field of heliophysics.
Hanna Holborn Gray Special Collections Research Center, University of Chicago Library
Introduction
At this point, we knew that solar magnetism was behaving in ways we weren’t expecting. SOHO data had revealed that globally, the solar magnetic field was far more variable than we had imagined. And the particles comprising the solar wind, as measured near Earth, had peculiar compositional patterns that didn’t make sense if the wind was emanating directly from the sun’s surface, as had been predicted. It seemed that some kind of magnetic activity in the solar atmosphere was producing that wind — and the corona’s heat — but we didn’t have the models to explain how it worked.
The discussions in the meeting were long and intense, but they laid the foundation for a key decision: There was an absolute need to make observations closer to the sun with a mission notionally called Solar Probe. A model of that spacecraft — a probe that could withstand the harshness of the near-solar environment — was at the front of the meeting room, and after four decades of thinking about it, we were going to make it a reality. In 2017, shortly after I joined NASA as the head of science, the agency renamed the mission after Eugene Parker, based on my recommendation. It was now Parker Solar Probe.
Touching the Sun
Eugene Parker watched as Parker Solar Probe launched from Cape Canaveral in 2018 and rumbled into the sky atop a Delta IV Heavy rocket. After the liftoff he thanked me for the honor of having his name on this spacecraft and added, in a rare moment of directness, that he only wished some of those bastards — colleagues who’d derided his ideas and almost cost him his career — were still alive to see this.
The spacecraft used Venus flybys to sling itself successively closer to the sun, and on April 28, 2021, it touched the corona for the first time. It was now the closest spacecraft to our star and the fastest human-made object ever launched. (In fact, last month it passed by the sun for the 18th time at a speed that would get you from Washington, D.C., to Los Angeles in about 20 seconds, and from the Earth to the moon in 36 minutes.)
As hoped, the spacecraft’s near-sun observations were groundbreaking for our understanding of coronal heating. The observations solved the issue by decoding magnetic signatures in the extremely near-sun solar wind — a key to learning how the coronal furnace works.
From near Earth, the solar wind looks like a turbulent fluid that is loosely related to the sun at only the largest scales. But from up close, its structure directly reflects the structures on the solar surface. Instead of being a disorganized fluid, the near-sun solar plasma whooshes outward in streamlets that often match the sizes of the convective supergranules on the sun’s surface — the cells around which magnetic fields concentrate, amplify and escape into the corona.
During each solar orbit, the spacecraft zoomed through those streamlets, and it found a telltale fingerprint of magnetic activity that permeated the plasma and pointed to a source for the corona’s heat. Called “switchbacks,” these fingerprints were S-shaped structures formed by brief reversals in the locally measured magnetic field. Such switchbacks form (at least, according to most scientists) when closed magnetic loops collide with open magnetic loops and connect with them, during what’s known as an interchange reconnection event. As with good champagne in a bottle, the only way to release energy and plasma from a tangled, closed magnetic loop is to uncork it by breaking it open and reconnecting it with an open field line. These reconnection events generate heat and sling solar material into space — thus warming the corona and accelerating particles in the solar wind.
Although some scientists aren’t completely convinced the problem is solved, the field is now converging on the conclusion that Parker’s 1988 explanation was right. Coronal heating ultimately depends on magnetic fields at small scales. Convective granules on the solar surface concentrate magnetic fields at their edges and unleash a chain of events that, through subsequent magnetic interactions in the atmosphere, leads to the supersonic solar wind and the million-degree temperatures we see.
Later this year, Parker Solar Probe will break its own record and fly even closer to the sun. Another trip to hell and back, in search of more answers to outstanding solar mysteries.
Quelle: Quanta Magazine
----
Update: 31.08.2024
.
Solar Orbiter shows how solar wind gets a magnetic push
ESA’s Solar Orbiter spacecraft has provided crucial data to answer the decades-long question of where the energy comes from to heat and accelerate the solar wind. Working in tandem with NASA’s Parker Solar Probe, Solar Orbiter reveals that the energy needed to help power this outflow is coming from large fluctuations in the Sun’s magnetic field.
The solar wind is a constant stream of charged particles that escapes from the solar atmosphere (called the corona) to flow out past Earth. It's the collision of the solar wind with our planet’s atmosphere that triggers the colourful aurora in our skies.
‘Fast’ solar wind moves with speeds above 500 km/s, equivalent to a whopping 1.8 million km/h. Curiously, this wind exits the Sun’s corona with lower speeds, so something speeds it up as it moves farther away. The million-degree wind naturally cools down as it expands into a larger volume and becomes less dense, much like the air on Earth as you climb a mountain. And yet, it cools more slowly than expected from this effect alone.
So, what provides the necessary energy to accelerate and heat the fastest parts of the solar wind? Data from ESA’s Solar Orbiter and NASA’s Parker Solar Probe have provided conclusive evidence that the answer is large-scale oscillations in the Sun’s magnetic field, known as Alfvén waves.
“Before this work Alfvén waves had been suggested as a potential energy source, but we didn’t have definitive proof,” says joint first author of the work Yeimy Rivera from the Center for Astrophysics, Harvard & Smithsonian, Massachusetts.

In an ordinary gas, such as Earth’s atmosphere, the only kind of waves that can be transmitted are sound waves. However, when a gas is heated to extraordinary temperatures, such as in the Sun’s atmosphere, it enters an electrified state known as a plasma and responds to magnetic fields. This allows waves, called Alfvén waves, to form in the magnetic field. These waves store energy and can efficiently carry it through a plasma.
A normal gas expresses its stored energy in the form of density, temperature and velocity. With a plasma, however, the magnetic field also stores energy. Both Solar Orbiter and Parker Solar Probe contain the necessary instruments to measure the properties of the plasma, including its magnetic field.
Although the two spacecraft are operating at different distances from the Sun, and in very different orbits, in February 2022, the spacecraft happened to align along the same stream of solar wind.
Parker, operating at 13.3 solar radii (around 9 million km) from the Sun at the very outer edges of the Sun’s corona, crossed the stream first. Solar Orbiter, operating at 128 solar radii (89 million km), then crossed the stream a day or two later. “This work was only possible because of the very special alignment of the two spacecraft that sampled the same solar wind stream at different stages of its journey from the Sun,” says Yeimy.
Taking full advantage of this rare alignment, the team compared the measurements of the same plasma stream at two different locations. They first transformed the measurements into four key energy quantities, which included a measurement of the stored energy in the magnetic field, called the wave energy flux.
Because energy can neither be created nor destroyed, only converted from one form to another, the team compared the readings from Parker to those from Solar Orbiter. They did this comparison both with and without the magnetic energy term.
“We found that if we didn't include the wave energy flux at Parker, we don't quite match how much energy we have at Solar Orbiter,” says joint first author Samuel Badman, Center for Astrophysics, Harvard & Smithsonian, Massachusetts.
Close to the Sun, where Parker measured the stream, around 10% of the total energy was found in the magnetic field. At Solar Orbiter, this number had dropped to just 1% but the plasma had accelerated and had cooled more slowly than expected.
Comparing the numbers, the team concluded that the lost magnetic energy was powering the acceleration and slowing down the cooling of the plasma by providing some heating of its own.

The data also show how important magnetic configurations known as switchbacks are to the acceleration of the wind. The switchbacks are large deflections in the Sun’s magnetic field lines and are examples of Alfvén waves. They have been seen since the first solar probes of the 1970s but their detection rate has dramatically increased since Parker Solar Probe became the first spacecraft to fly through the Sun’s corona in 2021 and detected that switchbacks join together in patches.
This new work confirms that these patches of switchbacks contain enough energy to be responsible for the missing portion of the acceleration and heating of the fast solar wind.
“This new work expertly brings together some large pieces of the solar puzzle. More and more, the combination of data collected by Solar Orbiter, Parker Solar Probe and other missions is showing us that different solar phenomena actually work together to build this extraordinary magnetic environment,” says Daniel Müller, ESA Project Scientist for Solar Orbiter.
And it’s not just telling us about our Solar System. “Our Sun is the only star in the Universe where we can directly measure its wind. So what we learned about our Sun potentially applies at least to other Sun-type stars, and perhaps other types of stars that have winds,” says Samuel.
The team is currently working on expanding their analysis to apply to slower forms of the solar wind, to see whether the Sun’s magnetic field energy plays a role in their acceleration and heating too.
Quelle: ESA
----
Update: 6.11.2024
.
Final Venus Flyby for NASA’s Parker Solar Probe Queues Closest Sun Pass
Parker’s Venus flybys have become boons for new Venus science thanks to a chance discovery from its Wide-Field Imager for Parker Solar Probe, or WISPR. The instrument peers out from Parker and away from the Sun to see fine details in the solar wind. But on July 11, 2020, during Parker’s third Venus flyby, scientists turned WISPR toward Venus in hopes of tracking changes in the planet’s thick cloud cover. The images revealed a surprise: A portion of WISPR’s data, which captures visible and near infrared light, seemed to see all the way through the clouds to the Venusian surface below.
“The WISPR cameras can see through the clouds to the surface of Venus, which glows in the near-infrared because it’s so hot,” said Noam Izenberg, a space scientist at the Johns Hopkins Applied Physics Laboratory in Laurel, Maryland.
Venus, sizzling at approximately 869 degrees Fahrenheit (about 465 C), was radiating through the clouds.
The WISPR images from the 2020 flyby, as well as the next flyby in 2021, revealed Venus’ surface in a new light. But they also raised puzzling questions, and scientists have devised the Nov. 6 flyby to help answer them.

The Venus images correspond well with data from the Magellan spacecraft, showing dark and light patterns that line up with surface regions Magellan captured when it mapped Venus’ surface using radar from 1990 to 1994. Yet some parts of the WISPR images appear brighter than expected, hinting at extra information captured by WISPR’s data. Is WISPR picking up on chemical differences on the surface, where the ground is made of different material? Perhaps it’s seeing variations in age, where more recent lava flows added a fresh coat to the Venusian surface.
“Because it flies over a number of similar and different landforms than the previous Venus flybys, the Nov. 6 flyby will give us more context to evaluate whether WISPR can help us distinguish physical or even chemical properties of Venus’ surface,” Izenberg said.
After the Nov. 6 flyby, Parker will be on course to swoop within 3.8 million miles of the solar surface, the final objective of the historic mission first conceived over 65 years ago. No human-made object has ever passed this close to a star, so Parker’s data will be charting as-yet uncharted territory. In this hyper-close regime, Parker will cut through plumes of plasma still connected to the Sun. It is close enough to pass inside a solar eruption, like a surfer diving under a crashing ocean wave.
“This is a major engineering accomplishment,” said Adam Szabo, project scientist for Parker Solar Probe at NASA’s Goddard Space Flight Center in Greenbelt, Maryland.
The closest approach to the Sun, or perihelion, will occur on Dec. 24, 2024, during which mission control will be out of contact with the spacecraft. Parker will send a beacon tone on Dec. 27, 2024, to confirm its success and the spacecraft’s health. Parker will remain in this orbit for the remainder of its mission, completing two more perihelia at the same distance.
Quelle: NASA