12.03.2020
The behavior of one of nature's humblest creatures is helping astronomers probe the largest structures in the universe.
The single-cell organism, known as slime mold (Physarum polycephalum), builds complex filamentary networks in search of food, finding near-optimal pathways to connect different locations. In shaping the universe, gravity builds a vast cobweb structure of filaments tying galaxies and clusters of galaxies together along faint bridges hundreds of millions of light-years long. There is an uncanny resemblance between the two networks: one crafted by biological evolution, and the other by the primordial force of gravity.
The cosmic web is the large-scale backbone of the cosmos, consisting primarily of the mysterious substance known as dark matter and laced with gas, upon which galaxies are built. Dark matter cannot be seen, but it makes up the bulk of the universe's material. The existence of a web-like structure to the universe was first hinted at in the 1985 Redshift Survey conducted at the Harvard-Smithsonian Center for Astrophysics. Since those studies, the grand scale of this filamentary structure has grown in subsequent sky surveys. The filaments form the boundaries between large voids in the universe.
But astronomers have had a difficult time finding these elusive strands, because the gas is so dim it is hard to detect. Now a team of researchers has turned to slime mold to help them build a map of the filaments in the local universe (within 500 million light-years from Earth) and find the gas within them.
They designed a computer algorithm, inspired by slime-mold behavior, and tested it against a computer simulation of the growth of dark matter filaments in the universe. A computer algorithm is similar to a recipe that tells a computer precisely what steps to take to solve a problem.
The researchers then applied the slime mold algorithm to data containing the locations of 37,000 galaxies mapped by the Sloan Digital Sky Survey at distances corresponding to 300 million light-years. The algorithm produced a three-dimensional map of the underlying cosmic web structure.
They then analyzed the ultraviolet light from 350 quasars (at much farther distances of billions of light-years) cataloged in the Hubble Spectroscopic Legacy Archive, which holds the data from NASA's Hubble Space Telescope's spectrographs. These distant cosmic flashlights are the brilliant black-hole-powered cores of active galaxies, whose light shines across space and through the foreground cosmic web. Imprinted on that light was the telltale absorption signature of otherwise undetected hydrogen gas that the team analyzed at specific points along the filaments. These target locations are far from the galaxies, which allowed the research team to link the gas to the universe's large-scale structure.
"It's really fascinating that one of the simplest forms of life actually enables insight into the very largest-scale structures in the universe," said lead researcher Joseph Burchett of the University of California (UC), Santa Cruz. "By using the slime-mold simulation to find the location of the cosmic web filaments, including those far from galaxies, we could then use the Hubble Space Telescope's archival data to detect and determine the density of the cool gas on the very outskirts of those invisible filaments. Scientists have detected signatures of this gas for several decades, and we have proven the theoretical expectation that this gas comprises the cosmic web."
The survey further validates research that denser regions of intergalactic gas is organized into filaments that the team found stretches over 10 million light-years from galaxies. (That distance is more than 100 times the diameter of our Milky Way galaxy.)
The researchers turned to slime mold simulations when they were searching for a way to visualize the theorized connection between the cosmic web structure and the cool gas detected in previous Hubble spectroscopic studies.
Then team member Oskar Elek, a computational media scientist at UC Santa Cruz, discovered online the work of Sage Jenson, a Berlin-based media artist. Among Jenson's works were mesmerizing artistic visualizations showing the growth of a slime mold's tentacle-like network of food-seeking structures. Jenson's art was based on outside scientific research, which detailed an algorithm for simulating the growth of slime mold.
The research team noted a striking similarity between how the slime mold builds complex filaments to capture new food, and how gravity, in shaping the universe, constructs the cosmic web strands between galaxies and galaxy clusters.
Based on the simulation, Elek developed a three-dimensional computer model of the buildup of slime mold to estimate the location of the cosmic web's filamentary structure.
Although using a slime-mold-inspired simulation to pinpoint the universe's largest structures may sound bizarre at first, scientists have used computer models of these humble microorganisms, as well as grown them in petri dishes in a lab, to solve such complex problems as finding the most efficient traffic routes in large cities, solving mazes and pinpointing crowd evacuation routes. "These are hard problems to solve for a human, let alone a computer algorithm," Elek said.
"You can almost see, especially in the map of galaxies in the local universe from the Sloan data, where the filaments should be," Burchett explained. "The slime-mold model fits that intuition impressively. The structure that you know should be there is all of a sudden found by the computer algorithm. There was no other known method that was well suited to this problem for our research."
The researchers say that it is very difficult to design a reliable algorithm for finding the filaments in such a large survey of galaxies. "So it's quite amazing to see that the virtual slime mold gives you a very close approximation in just minutes," Elek explained. "You can literally watch it grow." Just for comparison, growing the organism in a petri dish takes days. Slime mold actually has a very special kind of intelligence for solving this one spatial task. After all, it's critical to its survival.
Quelle: NASA
+++
Astronomers use slime mold model to reveal dark threads of the cosmic web
The problem-solving prowess of a simple slime mold has been harnessed to trace the large-scale structure of the universe
A computational approach inspired by the growth patterns of a bright yellow slime mold has enabled a team of astronomers and computer scientists at UC Santa Cruz to trace the filaments of the cosmic web that connects galaxies throughout the universe.
Their results, published March 10 in Astrophysical Journal Letters, provide the first conclusive association between the diffuse gas in the space between galaxies and the large-scale structure of the cosmic web predicted by cosmological theory.
According to the prevailing theory, as the universe evolved after the big bang, matter became distributed in a web-like network of interconnected filaments separated by huge voids. Luminous galaxies full of stars and planets formed at the intersections and densest regions of the filaments where matter is most concentrated. The filaments of diffuse hydrogen gas extending between the galaxies are largely invisible, although astronomers have managed to glimpse parts of them.
None of which seems to have anything to do with a lowly slime mold called Physarum polycephalum, typically found growing on decaying logs and leaf litter on the forest floor and sometimes forming spongy yellow masses on lawns. But Physarumhas a long history of surprising scientists with its ability to create optimal distribution networks and solve computationally difficult spatial organization problems. In one famous experiment, a slime mold replicated the layout of Japan’s rail system by connecting food sources arranged to represent the cities around Tokyo.
Slime mold algorithm
Joe Burchett, a postdoctoral researcher in astronomy and astrophysics at UC Santa Cruz, had been looking for a way to visualize the cosmic web on a large scale, but he was skeptical when Oskar Elek, a postdoctoral researcher in computational media, suggested using a Physarum-based algorithm. After all, completely different forces shape the cosmic web and the growth of a slime mold.
But Elek, who has always been fascinated by patterns in nature, had been impressed by the Physarum “biofabrications” of Berlin-based artist Sage Jenson. Starting with the 2-dimensional Physarum model Jenson used (originally developed in 2010 by Jeff Jones), Elek and a friend (programmer Jan Ivanecky) extended it to three dimensions and made additional modifications to create a new algorithm they called the Monte Carlo Physarum Machine.
Burchett gave Elek a dataset of 37,000 galaxies from the Sloan Digital Sky Survey (SDSS), and when they applied the new algorithm to it, the result was a pretty convincing representation of the cosmic web.
“That was kind of a Eureka moment, and I became convinced that the slime mold model was the way forward for us,” Burchett said. “It’s somewhat coincidental that it works, but not entirely. A slime mold creates an optimized transport network, finding the most efficient pathways to connect food sources. In the cosmic web, the growth of structure produces networks that are also, in a sense, optimal. The underlying processes are different, but they produce mathematical structures that are analogous.”
Elek also noted that “the model we developed is several layers of abstraction away from its original inspiration.”
Of course, a strong visual resemblance of the model results to the expected structure of the cosmic web doesn’t prove anything. The researchers performed a variety of tests to validate the model as they continued to refine it.
Dark matter
Until now, the best representations of the cosmic web have emerged from computer simulations of the evolution of structure in the universe, showing the distribution of dark matter on large scales, including the massive dark matter halos in which galaxies form and the filaments that connect them. Dark matter is invisible, but it makes up about 85 percent of the matter in the universe, and gravity causes ordinary matter to follow the distribution of dark matter.
Burchett’s team used data from the Bolshoi-Planck cosmological simulation—developed by Joel Primack, professor emeritus of physics at UC Santa Cruz, and others—to test the Monte Carlo Physarum Machine. After extracting a catalog of dark matter halos from the simulation, they ran the algorithm to reconstruct the web of filaments connecting them. When they compared the outcome of the algorithm to the original simulation, they found a tight correlation. The slime mold model essentially replicated the web of filaments in the dark matter simulation, and the researchers were able to use the simulation to fine-tune the parameters of their model.
“Starting with 450,000 dark matter halos, we can get an almost perfect fit to the density fields in the cosmological simulation,” Elek said.
Burchett also performed what he called a “sanity check,” comparing the observed properties of the SDSS galaxies with the gas densities in the intergalactic medium predicted by the slime mold model. Star formation activity in a galaxy should correlate with the density of its galactic environment, and Burchett was relieved to see the expected correlations.
Now the team had a predicted structure for the cosmic web connecting the 37,000 SDSS galaxies, which they could test against astronomical observations. For this, they used data from the Hubble Space Telescope’s Cosmic Origins Spectrograph. Intergalactic gas leaves a distinctive absorption signature in the spectrum of light that passes through it, and the sight-lines of hundreds of distant quasars pierce the volume of space occupied by the SDSS galaxies.
“We knew where the filaments of the cosmic web should be thanks to the slime mold, so we could go to the archived Hubble spectra for the quasars that probe that space and look for the signatures of the gas,” Burchett explained. “Wherever we saw a filament in our model, the Hubble spectra showed a gas signal, and the signal got stronger toward the middle of filaments where the gas should be denser.”
In the densest regions, however, the signal dropped off. This too matched expectations, he said, because heating of the gas in those regions ionizes the hydrogen, stripping off electrons and eliminating the absorption signature.
“For the first time now, we can quantify the density of the intergalactic medium from the remote outskirts of cosmic web filaments to the hot, dense interiors of galaxy clusters,” Burchett said. “These results not only confirm the structure of the cosmic web predicted by cosmological models, they also give us a way to improve our understanding of galaxy evolution by connecting it with the gas reservoirs out of which galaxies form.”
Creative coding
Burchett and Elek met through coauthor Angus Forbes, an associate professor of computational media and director of the UCSC Creative Coding lab in the Baskin School of Engineering. Burchett and Forbes had begun collaborating after meeting at an open mic night for musicians in Santa Cruz, focusing initially on a data visualization app, which they published last year.
Forbes also introduced Elek to the work of Sage Jenson, not because he thought it would apply to Burchett’s cosmic web project, but because “he knew I was a nature pattern freak,” Elek said.
Coauthor J. Xavier Prochaska, a professor of astronomy and astrophysics at UCSC who has done pioneering work using quasars to probe the structure of the intergalactic medium, said, “This creative technique and its unanticipated success highlight the value of interdisciplinary collaborations, where completely different perspectives and expertise are brought to bear on scientific problems.”
Forbes’ Creative Coding lab combines approaches from media arts, design, and computer science. “I think there can be real opportunities when you integrate the arts into scientific research,” Forbes said. “Creative approaches to modeling and visualizing data can lead to new perspectives that help us make sense of complex systems.”
In addition to Burchett, Elek, Prochaska, and Forbes, the coauthors include Nicolas Tejos at the Pontifical Catholic University of Valparaiso, Chile; Todd Tripp at the University of Massachusetts, Amherst; and Rongmon Bordoloi at North Carolina State University. This work was supported by NASA.
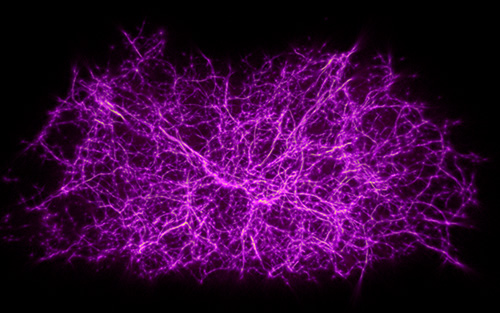
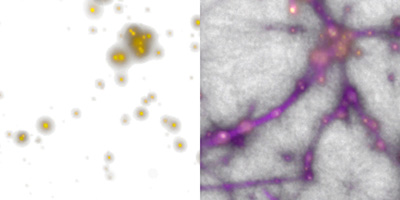
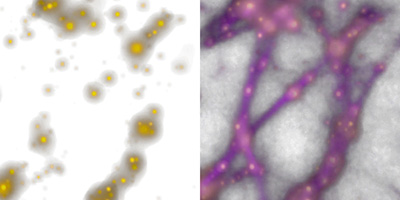
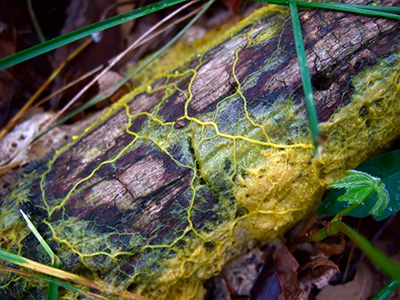
Quelle: THE UNIVERSITY OF CALIFORNIA