27.09.2016
-
LIVING WITH A COMET: A ROSINA TEAM PERSPECTIVE
Rosetta’s ROSINA (Rosetta Orbiter Spectrometer for Ion and Neutral Analysis) suite of instruments have made plenty of big headlines over the last two years, with the surprising discovery of molecular oxygen and nitrogen and the ‘flavour’ of the comet’s water being different to Earth’s among them. ROSINA principal investigator Kathrin Altwegg and colleagues reflect on the highs and lows of the 40-strong team of scientists and technicians since Rosetta woke up from deep space hibernation on 20 January 2014.
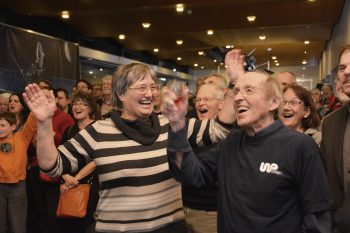
Celebrating Rosetta's wake up at the University of Bern, 20 January 2014. Image courtesy K. Altwegg.
Things actually did not get off to a particularly good start for us: all three of our sensors gave us error event messages when we first switched them on in the April after hibernation exit. There was a flurry of emails from the operations team on duty at the time querying just how unexpected these events were supposed to be. It turned out that the problem was due to the detector temperature of the DFMS [Double Focusing Mass Spectrometer]: it was at -30.8°C while the limit for operation is -30°C!
In addition, one of the RTOF [Reflectron Time-of-Flight] parameters was wrongly set and COPS [Comet Pressure Sensor] was too cold causing wrong offset values. The problems were solved three weeks later by a software patch and turning the spacecraft such that DFMS could bathe in the Sun and warm up.
Once we arrived at the comet on 6 August, and from then on, we could clearly see the comet in COPS data and in our mass spectra, which showed the rotation of the comet and the location of Rosetta over the northern (summer) and southern (winter hemisphere).
ROSINA research scientist Myrtha Hässig recalls she was trying on some clothes in a store in downtown San Antonio on a Saturday afternoon that August, when she got a call from her boss saying she should immediately look at the ROSINA data because ‘they are strange’. Myrtha said this phone call resulted in getting no new clothes, but instead the first detection of the coma heterogeneity! The result was reported in Science (and on this blog) in January 2015.
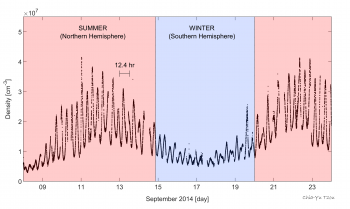
Early COPS data, which showed the rotation of the comet and the location of Rosetta over the northern (summer) and southern (winter hemisphere). Image courtesy K. Altwegg.
It was in October 2014, when Rosetta was 10 km from the comet surface, that ROSINA made the first detections of nitrogen and argon in the comet’s coma.
We could almost have concluded the mission there! With the deuterium in water, noble gas and nitrogen and a lot of organics detected we had already reached our major goals for this mission. It made us really proud to have such beautiful instruments – thank you to everyone who helped built them!
It was also in October that we blogged about the ‘perfume’ of the comet. The headline: “The comet smells of horse manure” was used in a Swiss Farmers newspaper, and was the first time a scientific space mission was reported in this newspaper
I remember talking to Martin Hilchenbach after I had first presented the ‘scent’ of the comet during a Rosetta team meeting in the September. He said: “What you have told us this morning about all the smelly stuff from the comet tells me that the comet is not habitable. For that it should at least have some alcohol to drink”. I then looked for ethanol in the data…and there it was! So 67P is habitable!
In another ROSINA team meeting Martin Rubin told me: “There is a peak on mass 23”. I told him that could not be possible, because only sodium has mass 23 and that’s a refractory. We never saw any signal on mass 23 during the whole Giotto mission, not one single count. Martin replied: “but there are also peaks on mass 28, 29, 30, 39, 40. Could be silicon and potassium”. Then Peter Wurz responded: “Wow! Must be solar wind sputtering, we always postulated this, but probably nobody believed it!’” (Read our blog write up about that here).
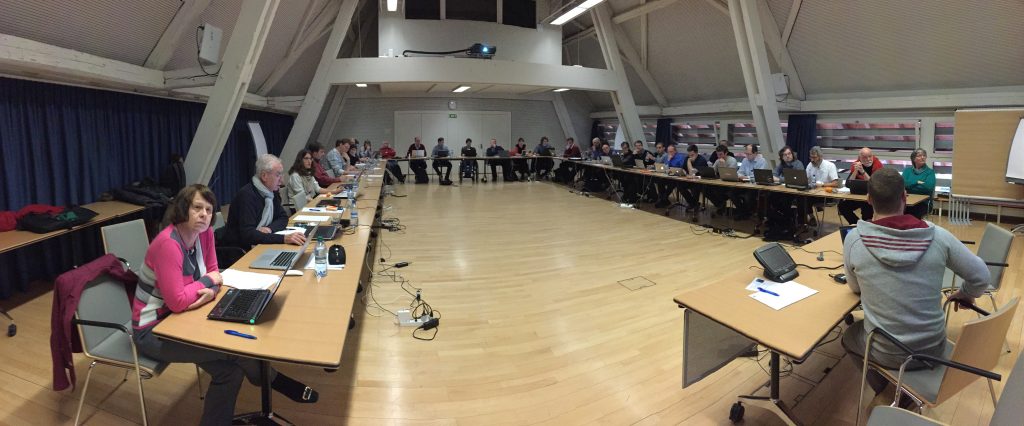
A ROSINA Co-Investigator meeting – there's always a lot of data to talk about...! Image courtesy K. Altwegg.
On another occasion when I needed a lot of convincing Martin told me: “We have a strange oxygen peak in the DFMS spectra. Could this be cometary?” I said: of course not, it is well known that O2cannot survive in the early Solar System as it is far too reactive. But it’s a bad sign. We probably have a major source of contamination. Let’s wait and see if it gets smaller. Two months later another colleague reported “the oxygen signal is still there, even higher than before and it varies with the cometary distance.” At this point I conceded that it did indeed look cometary, but I still needed convincing. It simply couldn’t be, could it? Six months later, another colleague again tells me “We have to publish the O2 immediately. I heard that Alice [another instrument on Rosetta] is also detecting O2.” But I still wanted to wait until after equinox to be completely sure…. In the end we published, in Nature, in October 2015.”
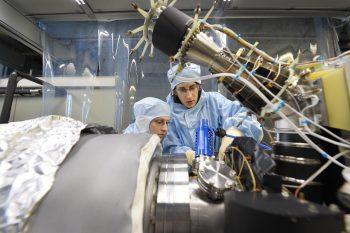
Ursina Calmonte (right) and Myrtha Hässig (left) with the ROSINA DFMS and the comet simulator CASYMIR. Photo by T. Wuethrich.
After this initial discovery period, and after the operations around Philae’s landing, the spacecraft was back at about 30 km distance from the comet for a while. We were not so happy because without so much comet activity at that time we couldn’t make such good measurements as we had in October, from 10 km. But then came the close 6 km flyby in February 2015. Again we had a bad start, with COPS misbehaving, giving pressure alerts and switching off the mass spectrometers. But just in time for the closest approach, the sensors start working again and showed very nice organic material from cometary dust!
Then, in March, the spacecraft had a safe mode, during another close flyby, forcing it far from the comet again. In the end this turned out to be a good thing for ROSINA. Even though we were far from the comet, we were still ‘feeling’ the cometary coma. The comet fought back, it showed us that it is unpredictable and that planning has to be flexible around such a body.
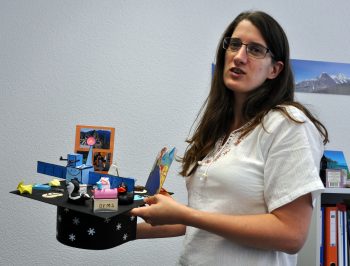
ROSINA PhD graduate Dr Ursina Calmonte with the thesis: “Sulfur Isotopic Ratios at 67P/Churyumov-Gerasimenko and Characterization of ROSINA-DFMS FM and FS", with her 'PhD hat' made by the ROSINA team. Image courtesy K. Altwegg.
As the comet became more active around perihelion, then ROSINA enjoyed some outbursts, and detected lots of organics. Additional detections of glycine were made during this time of increased activity – it had first been seen in October 2014, 10 km from the comet, and during the March flyby, 30-15 km from the comet. The first time I spotted glycine in the data I was on a 9-hour train journey and didn’t know quite what I was looking at at first. I wish I had paid more attention in chemistry lectures! The spectra were very complicated and I was trying to work out which molecules fit. I remember thinking “Oh, that seems to be glycine, an amino acid!”
Soon after perihelion, Rosetta went on a far excursion some 1500 km from the nucleus, to study the coma at large, and especially to explore the plasma environment further from the nucleus. There wasn’t so much for ROSINA to measure during such far excursions, but we used this time to do calibration work in the lab on the twin instruments.
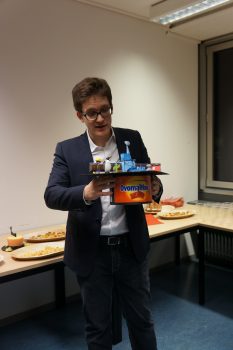
ROSINA PhD graduate Dr Sébastien Gasc (a chocolate addict!) with the thesis: “Sensitivity and Fragmentation Calibration of the ROSINA Reflectron-type Time-of-Flight Mass Spectrometer” – with his PhD hat made by the ROSINA team. Image courtesy K. Altwegg.
There was often some quite intense discussion – ‘fighting’ – within the Science Working Team (SWT) to put the spacecraft on the best trajectory or position with respect to the comet to get the best science for each instrument. Some you win, some you lose. I had been asking for a long period of observation since a planning meeting in December 2015. I really wanted to try and measure krypton and xenon before the end of the mission, as they’re really important measurements for cosmogeology. But, we would have to look constantly at the nucleus for a long time, which was not optimal for some of the other instruments. I had to be realistic, and balance political reality versus scientific needs, and determined that three weeks as close as possible to the comet, nadir-looking, would do the trick. And it did! The SWT agreed to implement three weeks in a row of priority time in May 2016, something we had waited for during the whole mission. And on the very first day we detected krypton and xenon! By the end of the three weeks we had just enough statistics to be able to make significant statements about the abundances of the noble gases as well as their isotopic ratios (the analysis of which is ongoing). What a feast! Thanks to all the other instrument teams who clearly were not happy during this time!
In the last month, Rosetta has been getting much closer to the comet again. We’re really excited about the final descent, as it will give us uninterrupted data from about 20 km to the impact point – we’ll get an unprecedented profile for the coma structure.
It will be a beautiful end to an exciting, but also very intense mission.
---
ROSETTA MEASURES PRODUCTION OF WATER AT COMET OVER TWO YEARS
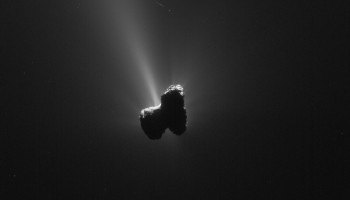
Comet 67P/C-G on 11 September 2015. Credit: ESA/Rosetta/NavCam – CC BY-SA 3.0
Over the past two years, Rosetta has kept a close eye on many properties of Comet 67P/Churyumov-Gerasimenko, tracking how these changed along the comet's orbit. A very crucial aspect concerns how much water vapour a comet releases into space, and how the water production rate varies at different distances from the Sun. For the first time, Rosetta enabled scientists to monitor this quantity and its evolution in situ over two years.
In a new study led by Kenneth C. Hansen of the University of Michigan, in the US, measurements of water production rate based on data from ROSINA, the Rosetta Orbiter Spectrometer for Ion and Neutral Analysis, are compared with water measurements from other Rosetta instruments.
The combination of all instruments shows an overall increase of the production of water, from a few tens of thousands of kg per day when Rosetta first reached the comet, in August 2014, to almost 100,000,000 kg per day around perihelion, the closest point to the Sun along the comet's orbit, in August 2015. In addition, ROSINA data show that the peak in water production is followed by a rather steep decrease in the months following perihelion.
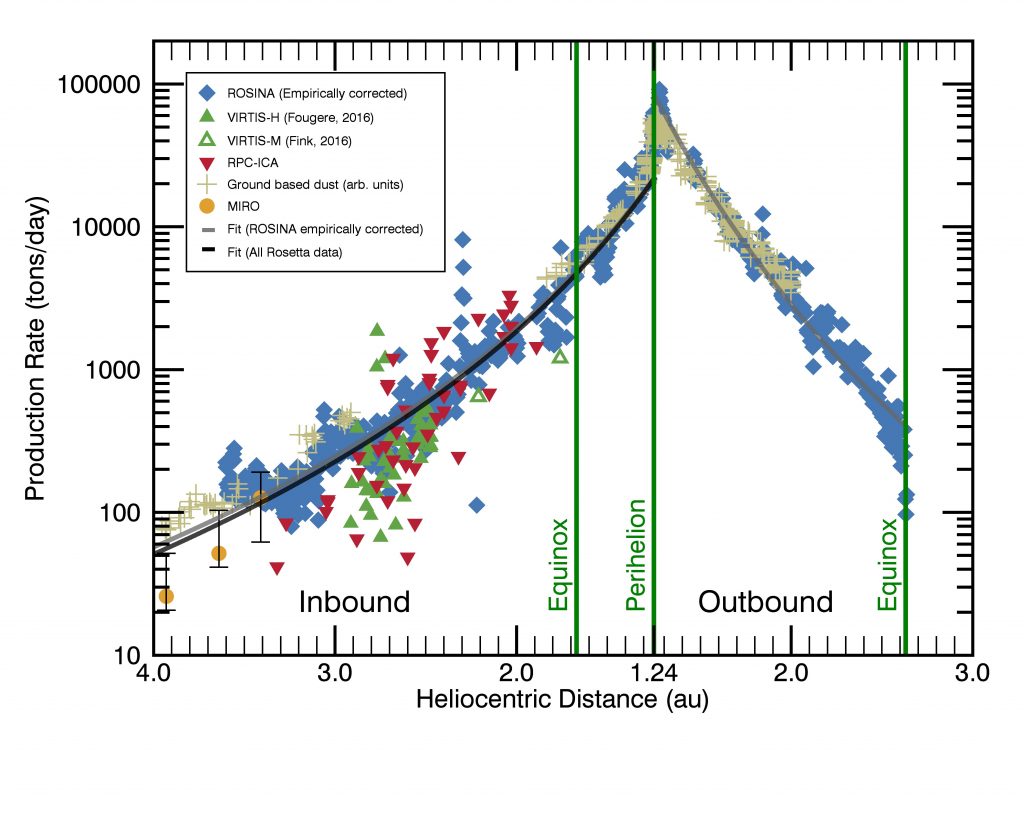
The water production rate measured at Comet 67P/C-G as a function of the comet's distance from the Sun (in astronomical units, au). The measurements are from ROSINA-DFMS (blue diamonds); MIRO (yellow circles); VIRTIS-H (solid green triangles); VIRTIS-M (unfilled green triangles); RPC-ICA (red triangles). The dust production rate, estimated from ground-based observations, is indicated in tan crosses. The data span the period between June 2014 and May 2016. Image adapted from Hansen et al (2016).
“We were pleasantly surprised to find such a good agreement between the data collected by all the various instruments in this unprecedented study of the water production rate's evolution for a Jupiter-family comet,” says Hansen.
The scientists analysed almost two years' worth of data from ROSINA, which detects neutral water molecules with its Double-Focussing Mass Spectrometer (DFMS).
“This is by no means trivial: ROSINA performs measurements locally, at specific points around the comet, and we need a model to extend them to the entire atmosphere,” adds Hansen.
The simplest model would be a spherical distribution of the outgassing centred around the nucleus but, given the complex shape and season cycle of Comet 67P/C-G, this would be a very crude approximation. For this reason, the ROSINA team developed a series of numerical simulations to accurately describe the comet's production of water, which are presented in a separate study led by Nicolas Fougere also of the University of Michigan.
From these simulations, which showed that the water production rate at a comet like 67P/C-G is highly inhomogeneous, Hansen and his colleagues derived an empirical model, which they then used to transform the local ROSINA measurements into estimates of the overall water production rate.
The results revealed that, during the first several months of observations, when the comet was at distances between 3.5 and 1.7 astronomical units (au) from the Sun, water was predominantly produced in the comet's northern hemisphere.
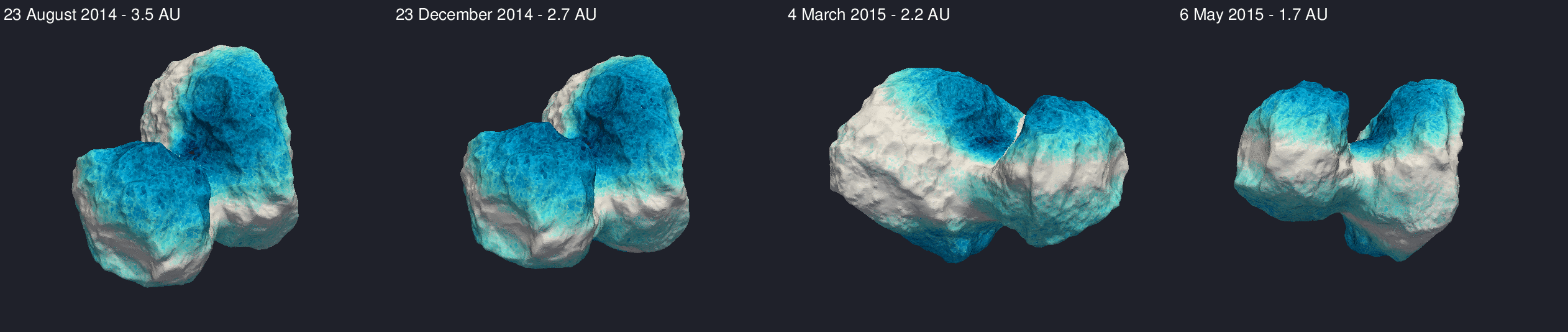
The water production rate predicted the simulations as Comet 67P/C-G approached the Sun, from August 2014 (left) to May 2015 (right), before the equinox that marked the end of the northern summer. Images adapted from Hansen et al (2016); animations courtesy of K.C. Hansen.
Then, in May 2015, the equinox marked the end of the 5.5-year long northern summer and the beginning of the short and intense southern summer. At that time, the comet was about 1.7 au from the Sun, and scientists expected that the peak of water production would drift slowly from the northern to the southern hemisphere; instead, this transition happened more abruptly than predicted. This was likely due to the complex shape of the nucleus, which causes highly variable illumination conditions including self-shadowing effects.
As expected, the production of water peaked between the end of August and early September 2015, about three weeks after the comet's perihelion, which took place on 13 August, 1.24 au from the Sun. The data hint at possible variations in the water production rate at this epoch: these might be due to the spacecraft's motion relative to the comet, but could also be an indication of actual changes to the outgassing dynamics, and will be subject of future in-depth investigation.
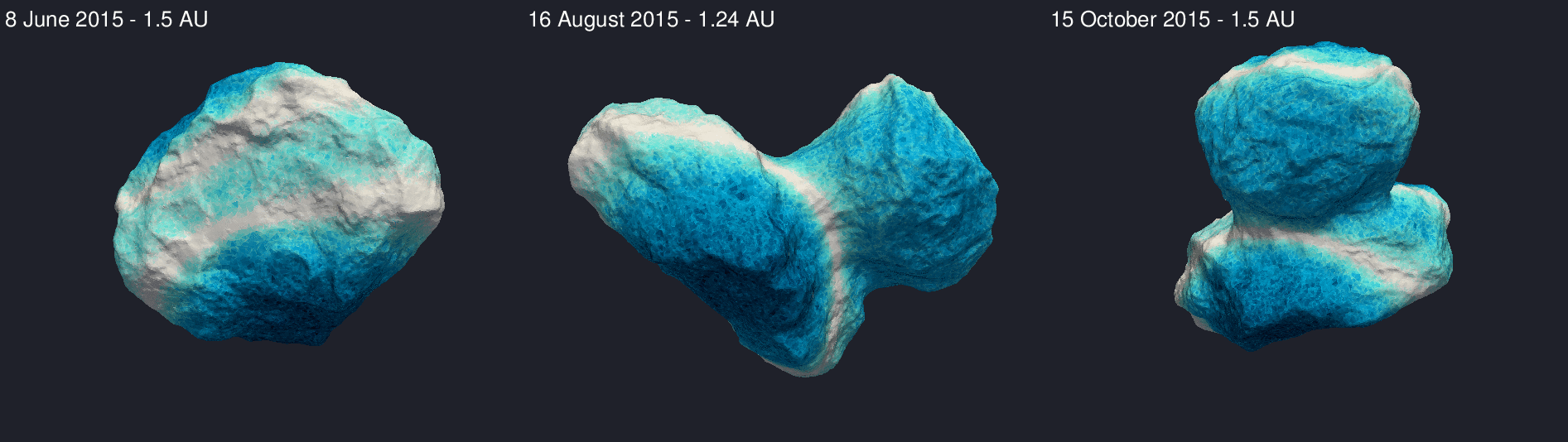
The water production rate predicted by simulations after the equinox of Comet 67P/C-G, which marked the beginning of the southern summer, and covering several weeks around the comet's perihelion. Images adapted from Hansen et al (2016); animations courtesy of K.C. Hansen.
In addition to the ROSINA measurements, Hansen and his colleagues collated a series of previously published measurements of the water production rate at 67P/C-G. These include observations performed with the Microwave Instrument for the Rosetta Orbiter (MIRO) shortly before and after Rosetta had reached the comet, data from the Visible and Infrared Thermal Imaging Spectrometer (VIRTIS) obtained between November 2014 and January 2015, and measurements from the Ion Composition Analyser, part of the Rosetta Plasma Consortium (RPC) suite of instruments, obtained between October 2014 and April 2015.
RPC-ICA does not detect water directly, but rather measures the ratio of differently ionised Helium ions; since He+ ions arise mainly from collisions between alpha particles (He2+) from the solar wind and neutral molecules, such as water, found in the comet's atmosphere, this ratio can be used to estimate the amount of water produced at the comet.
Hansen and his collaborators have found some small discrepancies between the various data sets: for example, the measurements from ROSINA yield systematically higher values than those from VIRTIS. One possible reason for this is the different nature of the two experiments: ROSINA samples the gas in the coma at the spacecraft's position, while VIRTIS tends to observe closer to the nucleus, where the water production activity is potentially more confined than it is further out in the coma. The difference in measurements techniques and the discrepancy could potentially indicate an extended source of water in the coma itself, for example icy grains that are lifted into the coma and turn into gas a few kilometers above the surface.
Another difference was found between the MIRO measurements, which indicate a rising trend in the water production rate from June to September 2014, and the first months of ROSINA data, starting in August, pointing to an almost constant rate in the same period.
“This could be explained if a sudden surge in the water production happened around the time of the first MIRO measurement, a few weeks before Rosetta's rendezvous with 67P/C-G, and the beginning of ROSINA observations,” says Hansen.
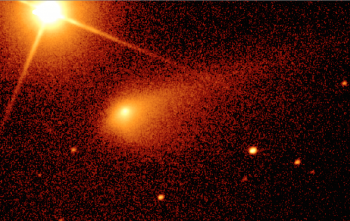
Comet 67P/C-G viewed from Earth on 30 September 2015. Credits: Alan Fitzsimmons / Liverpool Telescope
The scientists also compared the comet's production rate of water to that of dust, which can be measured via ground-based observations and was recently reported in a study led by Colin Snodgrass of the Open University, UK. These observations were performed with a number of robotic telescopes across the globe, from Chile to Hawaii and the Canary Islands.
“The correlation between the production rate of water and dust, both before and after perihelion, is impressive, suggesting that the gas-to-dust ratio remained constant over this long period,” explains Hansen.
Based on the water production rate, the team estimated that the comet lost some 6.4 billion kg of water to space over the period monitored by Rosetta, with the most intense mass loss happening near perihelion. The total mass loss, taking into account other gas molecules and in particular the dust, could be roughly 10 times larger than that and, if distributed uniformly across the comet nucleus, it would translate into a reduction of 2 to 4 metres.
“This study shows how cross comparison between different instruments and simulations is beginning to reveal the comet further,” says Matt Taylor, Rosetta project scientist at ESA.
“Connecting in-situ measurements from Rosetta with ground-based observations was a major science goal for the mission and it is wonderful to see this cooperation in action,” concludes Kathrin Altwegg, ROSINA principal investigator.
---
LIVING WITH A COMET: A MIRO TEAM PERSPECTIVE
Rosetta’s MIRO – the Microwave Instrument for the Rosetta Orbiter – has made nearly two billion science measurements at Comet 67P/C-G, and generated over 1.5 million spectra of gases in the comet’s coma. Principal Investigator Mark Hofstadter shares his team’s highlights of the mission, the challenges faced, and a hint of what’s still to come…
Being the first submillimeter instrument to fly in deep space, and having everything work are pretty high on our list of achievements! While we expected to be able to study the nucleus and coma gases with our instrument, and hoped to be able to see the dust particles, it was still a thrill to actually see the instrument doing what we thought it would. This comet puts out a lot of dust, and the dust particles are larger than predicted, so it was easier for MIRO to see the dust than we thought it would be, and we've been able to study it in more detail than anticipated.
But, just as for several of the instrument teams, the post-hibernation checkout gave us an initial unpleasant surprise. We were very cautious at first, and turned on one component of MIRO at a time, monitoring things in between to make sure everything was stable and working as expected. Early in the turn-on sequence, and near the end of a tracking pass, at JPL we saw that one of MIRO's sensors reported that the spectrometer's temperature shot up by 25 degrees for a few seconds, from about 20 to 45ºC. That could have been the sign of a short circuit temporarily heating things! Everything else looked normal to us, however, and the people in the control centre in Darmstadt said they saw nothing unusual. The original principal investigator of our instrument, Sam Gulkis, had just a few minutes to decide whether or not to command an immediate shut down of MIRO, before we lost radio contact with the spacecraft for several hours. What would you do? Shut down just in case something was short-circuiting to prevent further damage, or keep it on to avoid the risk of something having broken that would prevent us from turning it back on in the future? Sam, in Darmstadt, decided to leave MIRO on while we were out-of-contact. It was after midnight in Darmstadt when this happened, and Sam had been awake for many hours, so he went to sleep for a few hours while those of us at JPL (where it was still daytime) continued investigating. Sam later told us he fell asleep thinking we lost our instrument before even getting to the comet.
At JPL, one person asked the question “Why didn't the control centre in Darmstadt, who monitor all the instruments, warn us that they saw a temperature spike?” Instead, Darmstadt reported everything looked normal! Strangely, he found that we did not ask Darmstadt to monitor the particular spectrometer temperature that spiked. At the same time, a second person contacted the lead engineer for MIRO and began describing the situation to her. She said that she recalled that one of the temperature sensors we had was unreliable, and even on the ground before launch would sometimes give crazy readings. Things began to make sense! We looked back through our documents, and found that the temperature sensor that spiked was the unreliable one. Because we knew it was unreliable when we launched it in 2004, we told ESOC not to monitor it. But by 2014 we had forgotten the problem, and at JPL we were looking at all our temperature sensors and saw the (false) spike.
When Sam woke up, we were able to tell him that he made the right choice in leaving MIRO turned on, and when Rosetta came back in contact with the Earth, we saw that MIRO was operating normally with all temperatures and currents as expected.
This story highlights one of the challenges of a long duration mission like Rosetta – how do you remember important details about your instrument which was built years ago? Most of the instruments on Rosetta were designed in the mid-1990's, and started being built around 1997. We launched in 2004, and arrived at the comet in 2014. So now, near the end of 2016, we are using some hardware and software that is almost 20 years old. In many cases, the people who built it have retired. From the start, ESA realized that it was important to try and preserve as much human knowledge as possible in a format that would be easy to look up later. This included video documentation of the people building the instruments explaining anything that they thought could be relevant to the future, as well as typical user manuals and so-called design memos addressing very specific topics. MIRO’s user manual is about 300 pages long, and we are constantly updating it – we’re currently on version 5, and expect at least one more revision after the end of the mission!
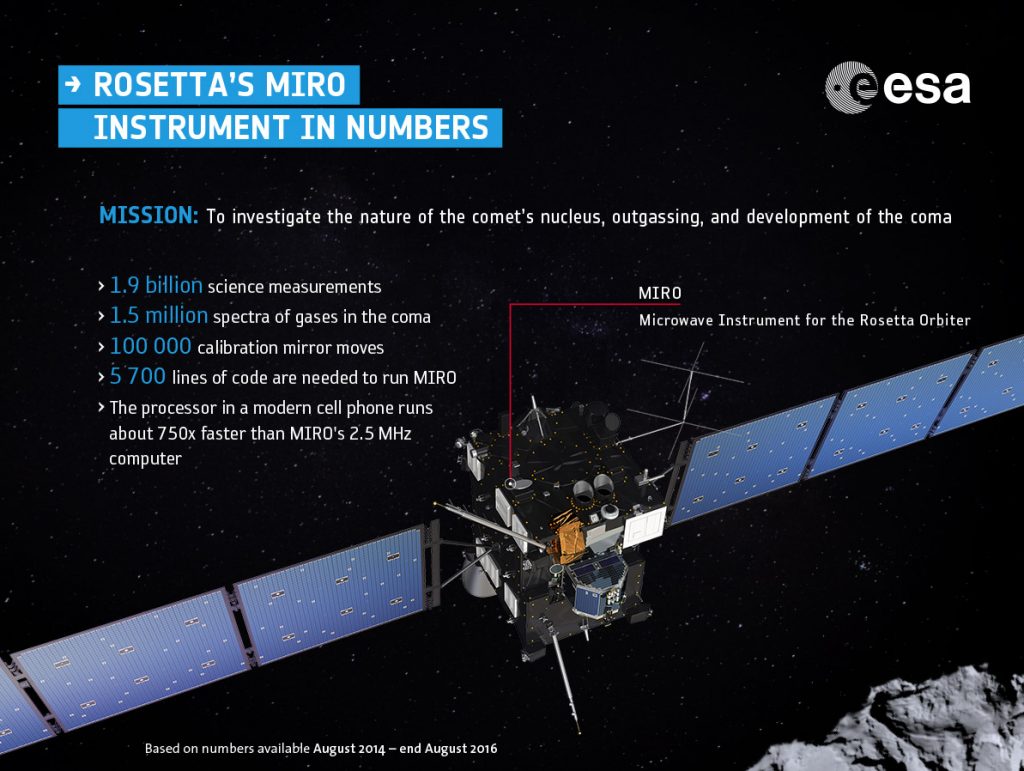
Mark adds that in addition to the above facts, there were also 7 children and 9 grandchildren born to members of the MIRO science team during Rosetta's mission.
Difficult detections
We were surprised at how difficult it was for MIRO to detect the gas CO (carbon monoxide) around the comet. CO interacts strongly with radio waves, and we expected it to be seen soon after water was detected. Instead, it was the last molecule we saw of the six MIRO was designed to measure. Water was seen early in the summer of 2014, while it was only near perihelion – in the summer of 2015 – that we saw CO. The ROSINA instrument had measured CO in certain areas around the comet before that, but it was not everywhere. That seems to be why MIRO (and some of the other instruments) had so much trouble detecting CO. Early in the mission we were spending most of our time in the northern hemisphere, while the CO was mostly coming from the southern hemisphere.
One of the most difficult observations we made at the comet was measuring the polarized emission from the surface. Polarization describes how the electric and magnetic fields in a radio wave are oriented. MIRO measures one of the two possible orientations of the radio waves, which is typically sufficient; most of the radio waves given off by the comet are unpolarized (meaning they have random orientations), so the total amount of radio energy emitted by the comet is twice what we measure. But when radio waves encounter an abrupt change – such as when they strike the boundary between the nucleus and space – at a certain angle, one polarization can be preferred over the other. The angle at which that occurs (called the Brewster Angle) can be used to determine some of the electrical properties of the nucleus material. By the way, polarized sunglasses use this principle: sunlight reflected off a roadway or the sea for example, is preferentially polarized in one direction, and the sunglasses block that polarization, reducing the glare. You can test the effects of polarization yourself: next time you are wearing polarized sunglasses compare what you see holding your head normally with what you see tilted 90 degrees to the side (so your ear is almost touching your shoulder); some reflections and some digital displays will look much dimmer with your head tilted one way or another.
MIRO wanted to take advantage of this effect by observing the same spot on the nucleus at about the same time, but with the spacecraft in two different orientations (just like tilting your head). That would allow MIRO to measure both possible polarizations of the radio waves, and any preference for one polarization over the other would let us learn something about the properties of the nucleus we could not figure out any other way. That turned out to be very difficult to do for the following reasons:
- It can take more than an hour to rotate the spacecraft 90 degrees. During that time, the spot we are looking at on the nucleus is moving and the viewing geometry is changing, making it hard to interpret our measurements.
- Because Rosetta is solar powered, we never want to tilt it in such a way as to prevent our solar panels from getting sunlight. That limits when we could try to make this measurement.
- Rotating the spacecraft can interfere with some of the measurements being made by other instruments on the spacecraft.
- Other high priority science investigations could only be done at the times when it was easiest to do our polarization measurements.
In the end, after lots of hard work from the Science Ground Segment folks at ESAC, they determined a handful of times we could make these measurements. They were successfully carried out, but it will take us quite awhile to fully calibrate and measure this small polarization effect.
Surprises and mysteries
Being surprised by what we see at the comet is, of course, one of the most fun things about this mission! MIRO has seen that some properties of the comet's nucleus (those related to how it warms and cools in response to sunlight) are relatively uniform all over the surface and down to a few centimetres beneath the surface. Many on our team expected these thermal properties to be much more variable. Other aspects of the nucleus, such as its composition, do seem to vary from place to place (such as the amount of CO, which I mentioned earlier). That is something we hoped to see, but were not sure whether or not it would be true. These are clues we are now using to try and figure out where and how comets form, and how they evolve over time.
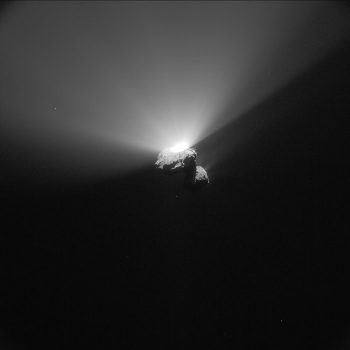
Comet outburst seen on 22 August 2015, during 67P/C-G's most active perihelion period. Credit: ESA/Rosetta/NavCam – CC BY-SA IGO 3.0
For me, one of the most surprising measurements was that some comet outbursts seem to have lots of dust but little gas. How can that be, given that we thought the gas is what lifts the dust? When ice in the nucleus sublimates (turns to gas), it expands rapidly into the relatively empty space around the comet. The gas moves at hundreds to over a thousand metres per second. This rapidly moving gas is what is thought to move most of the small dust particles seen lifted off the nucleus. Rosetta has observed many comet outbursts. In some of them, pictures reveal narrow ‘jets’ of small dust particles. Our expectation is that these dust jets are marking spots where gas jets exist. We were surprised to see that some dust jets don't seem to be associated with any extra gas emission, and similarly, we may have seen instances of gas jets existing without lifting any dust. Another mystery is that the MIRO instrument has seen the situation where a dust jet forms and the coma gases get 40ºC warmer, before the amount of gas increases (the 19 February 2016 outburst is the best studied example of this). What is going on?
The answer seems to be that Rosetta's close-up view is letting us see the effects of complex interactions among the gas, dust and the surface of the nucleus. We are still analysing the data, but some of the ideas being explored involve landslides on the nucleus that moves the dust (this allows dust emission to increase without any change in gas emission), and warm dust heating the relatively cool coma gases (explaining why the temperature of the gas could change even when the amount of gas did not).
Water, water everywhere…
MIRO observes changes in comet activity over time: this plot shows some comparisons in MIRO's measurements over the duration of the mission.
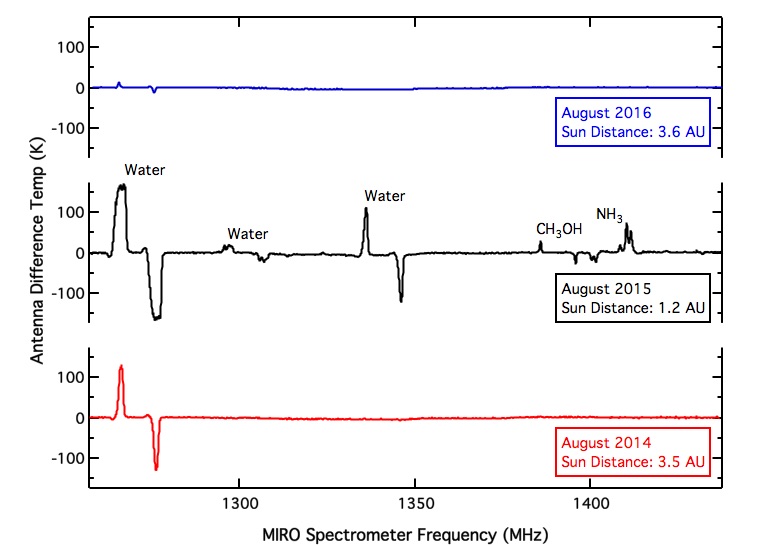
The bottom curve, in red, shows what MIRO measured in August 2014, when Rosetta first arrived at the comet and we were relatively far from the Sun (3.5 AU). The up and down spikes near the left edge of the plot are from water. By August 2015, when the comet was closest to the Sun (1.2 AU) and very warm, much larger amounts of water and other gases were detected (shown in the middle, black curve). The largest features are labeled with the molecule creating them: water (H2O), methanol (CH3OH), and ammonia (NH3). There are other species detectable whose signals are small and hard to see on this plot. By August 2016, the comet had moved away from the Sun again (3.6 AU) and cooled. The spectra at this time (blue curve at the top) shows only a small water line. In August 2016, the comet was emitting about 500x less water than it was in August of 2015. Graphic courtesy M. Hofstadter.
More science to come!
After the end of operations this month, we're looking forward to analysing everything, both because we know we're going to figure out all kinds of details when we have time to sit back and really think about our datasets, and because the most exciting things are probably going to be serendipitous discoveries! Recently MIRO has been studying how the nucleus properties change with depth in the upper tens-of-centimetres of the surface. Our simple models indicate there are changes, but we want to do more work to convince ourselves the effect is real. We’re also looking at how the presence of dust can influence gases far away from the dust, specifically what happens to the fast-moving gas as it moves through the much slower dust, and how heat emitted from the relatively warm dust might get absorbed by gas far away.
And finally…
Being part of this large, international team, doing something that is difficult and exciting, is a highlight of working this mission. On a personal note, it always gives me a feeling of pride in humanity to walk into one of our Rosetta science team meetings, and see how the individuals seamlessly transition among conversations internal to an instrument team to those across national or instrument boundaries. Languages and priorities may differ, but it is clear we are all working towards the success of the same mission.
This post is part of a series that looks behind the scenes of the instrument teams to find out what it was really like "living with a comet" for two years.
---
ROSETTA IN NUMBERS
Summary
Rosetta's mission lasted 12 years 6 months and 28 days, from launch on 2 March 2004 to mission end on 30 September 2016. During that time, the spacecraft travelled some 7.9 billion km, including three gravity assist flybys at Earth and one at Mars, and two asteroid flybys.
Travelling from Earth and eventually out towards the orbit of Jupiter, the vast distance between the spacecraft and the Sun, and spacecraft and Earth, has impacted on available solar power and data downlink capabilities respectively, as detailed in the graphic.
The majority of remaining figures shown apply to Rosetta's time at Comet 67P/Churyumov-Gerasimenko, between 6 August 2014 and the end of September 2016, although navigation camera images and science observations began again during the approach to the comet, following the spacecraft's wake up from deep space hibernation on 20 January 2014.
The total amount of (uncompressed) science data downlinked from Rosetta's instruments during its time at the comet is also shown. For definitions of instrument acronyms click here.
Graphic credit: ESA.
Quelle: ESA
-
Update: 28.09.2016
.
Gesellschaft für Lander Philae


Seit seiner erfolgreichen Landung am 12. November 2014 steht Lander Philae auf der Oberfläche des Kometen Churyumov-Gerasimenko. Nicht an der gemütlichen Stelle, die für seine Landung ausgewählt worden war, sondern nach drei Hüpfern dort, wo es rauh und schattig ist. Nun erhält der Lander auf dem Kometen allerdings Gesellschaft: Die Raumsonde Rosetta, die bisher um den Kometen kreiste, wird am 30. September 2016 ebenfalls auf Churyumov-Gerasimenko abgesetzt. Auch wenn die vierte Landung keine Landung im wörtlichen Sinne, sondern vielmehr ein sanfter Impakt ist - Orbiter und Lander werden dann wieder vereint auf dem Kometen um die Sonne reisen. "Natürlich ist es in gewisser Weise ein sentimentaler Anlass, wenn eine Mission, an der man über zwei Jahrzehnte gearbeitet hat, mit so einem Impakt endgültig zu Ende geht", sagt Philae-Projektleiter Dr. Stephan Ulamec vom Deutschen Zentrum für Luft- und Raumfahrt (DLR). "Andererseits haben wir so viele Daten gewonnen, dass man auch nicht traurig sein sollte."
Letzte Messungen, letzte Fotos
Der letzte Kontakt zu Philae fand am 9. Juli 2015 statt, für Rosetta wird die Kommunikation mit dem Aufschlagen auf dem Kometen enden. Doch zuvor wollen die Wissenschaftler möglichst langsam in Richtung Kometenoberfläche absinken, um währenddessen die letzten Messungen durchzuführen und die letzten Fotos aufzunehmen. Rosetta soll in der Ma’at-Region - ebenfalls auf dem Kopf des "entenförmigen" Kometen - ihre Reise beenden. "Ein besonders aktives Gebiet, mit Löchern, aus denen Gas und Staub ins All geschleudert wird", erläutert DLR-Wissenschaftler Stephan Ulamec. Vor über zwei Jahren, als Philae sich zur ersten Kometen-Landung überhaupt von der Rosetta-Sonde trennte, wäre exakt so ein Gebiet nicht erwünscht gewesen. "Wir wollten ja möglichst zielgenau an einem recht sicheren Ort landen". Dass es dann doch anders kam, weil die Harpunen des Landers diesen beim ersten Bodenkontakt nicht verankerten, war nicht geplant. Dennoch arbeitete das Team des DLR-Kontrollzentrums mit Philae - und konnten so 55 Stunden lang an dem unvorhergesehenen Standort wissenschaftliche Daten gewinnen. Erstmals wurde direkt auf einer Kometenoberfläche geschnüffelt, gehämmert, gebohrt und fotografiert.
Der Weg ist das Ziel
"Bei Rosetta ist aber vielmehr der Weg das Ziel", beschreibt der Philae-Projektleiter. Mehrere Instrumente sind nämlich während seines Abstiegs eingeschaltet - die Kunst ist es jedoch, rechtzeitig die letzten gewonnenen Daten vor dem Aufprall zur Erde hinunterzuladen. Noch rund 15 Sekunden vor dem Aufschlag - und damit dem Verlust der Kommunikation - soll das letzte Foto aufgenommen und zur Erde gesendet werden. Philae hatte im November 2014 seine Daten bis zur letzten Lebenssekunde seiner aufgeladenen Batterie gesendet und war dann bis zum Wiederaufladen mit Sonnenenergie in Winterschlaf gefallen. Bei seinen späteren Kontakten zum Bodenteam konnten nur wenige Daten empfangen werden. Wahrscheinlich wurden Philaes Sender durch die niedrigen Temperaturen in Mitleidenschaft gezogen, dies könnte die Ursache für die unregelmäßigen Kontakte und das anschließende Schweigen sein. Bei Rosetta hoffen alle, dass der Kontakt nicht frühzeitig abbricht und man so wertvolle Daten nicht mehr gesendet werden könnten. Alleine die Analyse der bisher gewonnenen Daten der ESA-Mission Rosetta wird die Wissenschaftler noch viele Jahre beschäftigen. "Die Resultate werden unser Bild von Kometen über viele Jahre prägen und unser Verständnis zur Entstehung des Sonnensystems und zu den Ursprüngen des Lebens vertiefen", ist sich Dr. Stephan Ulamec vom DLR sicher.
Mit Schrittgeschwindigkeit zum Abschied
Der Abschied von Rosetta wird ein langsamer sein: Seit dem 26. September 2016 sind die letzten dichten Überflüge beendet, und die Sonde wird auf ihren Abstieg vorbereitet. Fast 720 Millionen Kilometer wird Komet Churyumov-Gerasimenko von der Erde entfernt sein, wenn Rosetta gemächlich in Schrittgeschwindigkeit auf die Kometenoberfläche zufliegt. Gegen 13.20 Uhr mitteleuropäischer Zeit (mit einer Toleranz von plus/minus 20 Minuten) wird auf der Erde das Signal von Rosetta ausbleiben. Der Aufprall auf dem Kometen ist dann bereits Geschichte, denn das letzte Signal der Sonde hat eine Laufzeit von rund 40 Minuten.
Kometen und Asteroiden
Mit Rosettas Aufprall am 30. September 2016 findet eine Mission ihr Ende, die am 2. März 2004 ins All startete, deren Idee und Entwicklungszeit aber noch viel weiter zurückreichen. "Es ist wichtig, nun mehr über die kleinen Körper im Sonnensystem - also über Kometen und Asteroiden - zu lernen", sagt Dr. Stephan Ulamec vom DLR-Raumflugbetrieb. "Ohne Nachfolgemissionen würde die weltweit einzigartige Kompetenz zum Bau und Betrieb von Sonden wie Rosetta oder Land