.
Abstract
The Case for a Gaian Bottleneck: The Biology of Habitability
Aditya Chopra and Charles H. Lineweaver
The prerequisites and ingredients for life seem to be abundantly available in the Universe. However, the Universe does not seem to be teeming with life. The most common explanation for this is a low probability for the emergence of life (an emergence bottleneck), notionally due to the intricacies of the molecular recipe. Here, we present an alternative Gaian bottleneck explanation: If life emerges on a planet, it only rarely evolves quickly enough to regulate greenhouse gases and albedo, thereby maintaining surface temperatures compatible with liquid water and habitability. Such a Gaian bottleneck suggests that (i) extinction is the cosmic default for most life that has ever emerged on the surfaces of wet rocky planets in the Universe and (ii) rocky planets need to be inhabited to remain habitable. In the Gaian bottleneck model, the maintenance of planetary habitability is a property more associated with an unusually rapid evolution of biological regulation of surface volatiles than with the luminosity and distance to the host star. Key Words: Life—Habitability—Gaia—Abiogenesis habitable zone (AHZ)—Circumstellar habitable zone (CHZ). Astrobiology 16, 7–22.
-
1. Fermi’s Paradox, Hanson’s Great Filter, and Bostrom’s Bottlenecks
We see no evidence that our galaxy has been colonized by an advanced technological civilization. Archae- ological excavations have not unearthed alien spaceships, and
the optical and radio searches for extraterrestrial intelligence have not been successful (Tarter, 2001). If one assumes that once life emerges it evolves toward intelligence and techno- logical civilizations, we are faced with Fermi’s paradox: Where is everybody? [Webb (2002), C ́ irkovic ́ (2009); but also see Gray (2015) and Ward and Brownlee’s (2000) Rare Earth hypothesis]. To put this information in context, Hanson (1998) introduced the concept of a Great Filter, describing the pos- sible bottlenecks in the assumed progression from molecular chemistry to life, from life to intelligence, and from intelli- gence to galactic colonization. If the emergence of life is a rare and difficult process, then an emergence bottleneck could re- solve Fermi’s paradox. However, if technological civilizations inevitably destroy themselves, this self-destruction bottleneck could also resolve Fermi’s paradox.
Bostrom (2008) argued that the Great Filter is a valuable tool for assessing existential risks to humanity. If the biggest barrier in the Great Filter is the emergence bottleneck, then we will find no independently evolved life on Mars, and the apparent absence of advanced technological civilizations in
our galaxy is because life’s emergence is difficult and rare. In this case, humanity has already survived the biggest threat to its continued existence; the biggest bottleneck is behind us, and we can relax. However, if we find life on Mars that has evolved independently of life on Earth, this would be strong evidence that there is no emergence bottleneck. If we find such life, Bostrom argues that the biggest bottleneck—the self-destruction bottleneck—would then be in front of us. This would be ‘‘by far the worst news ever printed on a newspaper cover.’’ As a plausible alternative to such catastrophic logic, we introduce the concept of a Gaian bottleneck, a bottleneck that life on Earth has already passed through. If such a bot- tleneck exists, the discovery of extant independently evolved martian life might be bad news, but the discovery of extinct independently evolved martian life would not be.
In the standard view, the decrease in bombardment rate from *4.5 to *3.8Gya is associated with making Earth more clement and thus enabling life to emerge and persist (Maher and Stevenson, 1988). In contrast to this view, we postulate a Gaian bottleneck model in which early life (on Earth and elsewhere) is not just a passive passenger but comes under strong selection pressure to actively modify and regulate its environment. The emergence of life’s abilities to modify its environment and regulate initially abiotic feedback mecha- nisms (what we call Gaian regulation) could be the most sig- nificant factor responsible for life’s persistence on Earth.
This highlights an important difference between physics- based estimates of habitability and the more unpredictable patterns of biological evolution on the highly unstable sur- face environments of young terrestrial planets. For example, bombardment rates inevitably decrease in the circumstellar habitable zones (CHZs) of stars, but the timescales for the evolution of Gaian regulation are probably unpredictable and would not inevitably evolve rapidly (or at all). Thus, if there is anything special about what happened on Earth to allow life to persist here, it might have less to do with the decreasing bombardment rate in the Hadean, or special chemical ingredients, or sources of free energy, or even a rare recipe for the emergence of life. The existence of life on Earth today might have more to do with the unusually rapid biological evolution of effective niche construction and Gaian regulation in the first billion years. Habitability and habitable zones would then not only be a passive abiotic property of stellar and planetary physics and chemistry (such as stellar luminosity, initial water content, and de- creasing bombardment rate) but would also be a result of early life’s ability to influence initially abiotic geochemical cycles and turn them into the life-mediated biogeochemical cycles that we are familiar with on the current Earth (Len- ton, 1998; Lenton et al., 2004; Schneider, 2004; Falkowski et al., 2008; Kump et al., 2009). Without rapid evolution of Gaian regulation, early extinction would be the most com- mon fate of planetary life. Even if the emergence of life is a common feature of wet rocky planets throughout the Uni- verse, the Gaian bottleneck model suggests that inhabited Earth-like planets would be rare.
2. If There Is an Emergence Bottleneck, It Is Probably ‘‘Recipe-Based’’
2.1. No stellar bottleneck. No wet-rocky-planet bottleneck. Sun-like stars and Earth-like planets are common.
If our Sun were the most uranium-rich star in the Galaxy, and if life on Earth were uranium-based (instead of carbon- based), then we would have good reason to believe that life (either its emergence or persistence, or both) requires a rare kind of uranium-rich host star. However, we have been unable to identify any significant differences between the Sun and other stars that could plausibly be connected with an increased probability of abiogenesis. Sun-like stars are common in the Universe (Robles et al., 2008). Thus, there seems to be no stellar bottleneck responsible for reducing the probability for the emergence of life.
Over the past decade, estimates for the frequency of rocky planets in, or near, the CHZ have increased (Howard et al., 2012; Lineweaver and Chopra, 2012a; Bovaird and Line- weaver, 2013; Fressin et al., 2013; Petigura et al., 2013; Marcy et al., 2014; Bovaird et al., 2015; Burke et al., 2015). Rocky planets in the CHZ are likely to be a common outcome of planetary formation. This result is supported by observa- tional, theoretical, and computational models of rocky planet formation in which gas-rich protoplanetary disks evolve into dust disks in which planetesimals form and undergo oligar- chic growth into planetary embryos as they differentiate into iron-nickel-rich cores, silicate mantles, and crusts dominated by incompatible lithophiles (Elkins-Tanton, 2012; Morbidelli et al., 2012; Chambers, 2014; Hardy et al., 2015). Models and
observations suggest that this sequence—the formation of terrestrial planets—is not a rare occurrence that needs special initial conditions. There seems to be no rocky-planet-in-the- CHZ bottleneck responsible for reducing the probability for the emergence of life.
2.2. No elemental or molecular ingredient bottleneck.
Life on Earth is made of hydrogen, oxygen, carbon, ni- trogen, sulfur, and phosphorus: ‘‘HOCNSP’’ (Chopra et al., 2010). HOCNSP are among the most abundant atoms in the Universe (Pace, 2001; Lodders et al., 2009; Lineweaver and Chopra, 2012b). Since the elemental ingredients for life are the most common elements in the Universe, it is not surprising that the molecular ingredients of life are also common.
Of the elements in the Universe that form molecules, water (H2O) is the combination of the first and second most abun- dant elements. Water should be a common feature of rocky planets (Raymond et al., 2004, 2007; Elkins-Tanton, 2012). Radial mixing during the epoch of oligarchic growth ensures that some water-rich materials from beyond the snowline are injected into the more water-poor material of the feeding zones of rocky planets in the CHZ. Thus, it is likely that Mars and Venus both started out, like Earth, hot from accretional energy and impacts and wet from impacts of large hydrous (5– 20% water) asteroids from the outer asteroid belt ( Morbidelli et al., 2000, 2012) and other ‘‘wet’’ accretionary material (Drake and Righter, 2002; Drake, 2005). Terrestrial planets in other planetary systems are also likely to start with variable, non-negligible amounts of water.
Current terrestrial life is built of monomers such as amino acids, fatty acids, sugars, and nitrogenous bases. Amino acids link together to form proteins; fatty acids link to form lipids; sugars link to form carbohydrates; and nitrogenous bases combine with sugar and phosphate to make nucleotide mono- mers, which link to form RNA/DNA (Lineweaver and Chopra, 2012b). Thus, life on Earth emerged when available monomers linked together to make polymers. Amino acids and other or- ganic monomers fall from the skies in carbonaceous chondrites. We have no reason to believe that the availability of these monomers is somehow unique to Earth or the Solar System. The flux of such organics was particularly high during the first billion years of the formation of Earth, and we have no reason to believe that this will not be the case during the formation of terrestrial planets in other planetary systems. The assortment of organic compounds found in carbonaceous chondrites and the probable universality of early heavy meteoritic bombardments indicate that new planetary systems should also be supplied with organic ingredients and be conducive to the synthesis of prebiotic molecules (Ehrenfreund and Charnley, 2000; Herbst and van Dishoeck, 2009; Tielens, 2013). We expect all the ingredients of life as we know it (HOCNSP, water, amino acids, sugars, nucleic acids, HCN, and other organics) to be present and available on wet rocky planets throughout the Universe. An ingredient bottleneck seems implausible.
2.3. No free energy bottleneck since photon- and chemical redox–based energy
sources are common.
Life (here and elsewhere) needs to do something for a living (Conrad and Nealson, 2001; Nealson and Rye, 2013). This living depends on extracting free energy from an
environment out of thermodynamic equilibrium (Branscomb and Russell, 2013). This extraction is based on catalyzing redox reactions or absorbing photons (Kleidon, 2012). The interiors of rocky planets throughout the Universe are denser and hotter than their surfaces. Thus, thermal gradients, density gradients, and the fluid flows they drive, set up redox potentials that can be exploited (Nisbet and Fowler, 2014). The environmental factors that enabled abiogenesis on Earth, such as the geochemical disequilibria between rocks, minerals, aqueous species, and gases, are likely to be ubiquitous on wet rocky planets throughout the Universe. In addition, for planets in the CHZ, fusion in a nearby star shines *6000 K photons onto *300 K surfaces and enables metabolisms based on photon capture (Annila and Annila, 2008; Lineweaver and Egan, 2008).
2.4. Recipe bottleneck? How ubiquitous are abiogenesis habitable zones?
With the absence of bottlenecks associated with stars, planets, ingredients, and sources of free energy, the only other factor that could produce an emergence bottleneck would be ‘‘recipe.’’ While we do not know the specifics of the prebiotic chemistry and geochemical environments neces- sary for life to emerge (e.g., Orgel, 1998; Stu ̈eken et al., 2013), the view that life will emerge with high probability on Earth-like planets is shared by many scientists. Darwin (1871) speculated that the environment, ingredients, and energy sources needed for the origin of life could be common, when he wrote about life emerging in ‘‘some warm little pond with all sort of ammonia and phosphoric salts, light, heat, electricity present, that a protein compound was chemically formed, ready to undergo still more complex changes.’’
de Duve (1995) has been a more recent proponent of the view that the emergence of life is a cosmic imperative: ‘‘Life is either a reproducible, almost commonplace mani- festation of matter, given certain conditions, or a miracle. Too many steps are involved to allow for something in between.’’ Discarding miracles, de Duve leaves us with life as an ‘‘almost commonplace manifestation of matter.’’ As far as we know, Darwin’s warm little pond or de Duve’s ‘‘certain conditions’’ are common on Earth-like planets and may be sufficient for the emergence of life. Biota throughout the Universe would emerge from chemistry through proto- biotic molecular evolution (e.g., Eigen and Winkler, 1992; Eschenmoser and Volkan Kisaku ̈rek, 1996; Orgel, 1998; Ward and Brownlee, 2000; Martin and Russell, 2007; Russell et al., 2013).
There is some tension between this conclusion and the inability of synthetic biologists to produce life, despite having access to a wide variety of ingredients, environmental setups, and energy sources. Plausible explanations for this tension include (1) there is an emergence bottleneck due to a con- voluted recipe whose requirements only rarely occur natu- rally; or (2) there is no emergence bottleneck—the recipe for life is simple—but we are not as imaginative or as resourceful as nature, so we have not replicated the recipe in the relatively short time we have been investigating the origin of life. We conclude that the idea of an emergence bottleneck is still plausible. However, the evidence for it is getting weaker as we find out more about the proto-biotic molecular evolution that led to the emergence of life on Earth (e.g., Benner, 2013;
England, 2013; Nisbet and Fowler, 2014; Sousa and Martin, 2014). This weakness provides motivation for alternative explanations for the apparent paucity of life in the Universe. The Gaian bottleneck hypothesis is one such alternative.
As we learn more about the origin of life, we can begin to define an abiogenesis habitable zone (AHZ) where the re- quirements for life’s emergence are met. Many initially wet rocky planets in the CHZ may possess the necessary and sufficient conditions to get life started (AHZ in Fig. 1). The habitability requirements for the origin of life may be sub- stantially different from, and more specific than, the re- quirements to maintain life on a planet (as in the difference between the need to have a spark plug to start an engine and a radiator to keep it from overheating). In Fig. 1, we have assumed that the AHZ is not necessarily a subset of cur- rently inhabited planetary environments.
3. Emergence Bottlenecks versus Gaian Bottlenecks
An emergence bottleneck is illustrated in Fig. 2. The left panel shows a hypothetical planet with non-evolving plan- etary conditions. The right panel shows a more plausible planet that initially had some habitable regions but, through volatile evolution or other transient factors, lost its surface water and evolved away from habitable conditions (e.g., a runaway greenhouse or runaway glaciated planet). Without significant abiotic negative feedback mechanisms, the sur- face environments of initially wet rocky planets are volatile and change rapidly without any tendency to maintain the habitability that they may have temporarily possessed as their early unstable surface temperatures transited through habitable conditions (Fig. 6C).
If there is no emergence bottleneck (Fig. 3), typical wet rocky planets have initial conditions compatible with the emergence of life (AHZ). We postulate that almost all ini- tially wet rocky planets on which life emerges (left panel of Fig. 3) quickly evolve like the abiotic planets represented in the right panel of Fig. 2. This unregulated evolution of planetary environments away from habitable conditions constrains the duration of life’s existence on the planet. We call this early extinction of almost all life that ever emerges the Gaian bottleneck. In rare cases (for example on Earth), life will be able to evolve quickly enough to begin to reg- ulate surface volatiles through the modification of abiotic feedbacks (right panel of Fig. 3). The potentially relevant feedbacks involved in such early Gaian regulation are il- lustrated in Fig. 5 and discussed in Table 1 and Section 5.
4. Early Extinction from Impacts and Devolatilization
4.1. Bombardment, impact frustration, and extinction
During the late phases of Earth’s accretion (*4.5 to *4.0Gya), episodes of cold ‘‘Norse ice-hell’’ were punc- tuated by brief periods of hot inferno with magma oceans (Nisbet, 2002; Elkins-Tanton, 2008). Frequent, large, ran- dom impacts produced wide-ranging, unstable temperatures. The largest impacts were so large that any life that did emerge during the transitory habitable periods was probably bombarded into extinction. These early bombardment- induced extinctions have been called the impact frustration of life (Maher and Stevenson, 1988; Sleep et al., 1989, 2014; Davies and Lineweaver, 2005; Marchi et al., 2014).
.
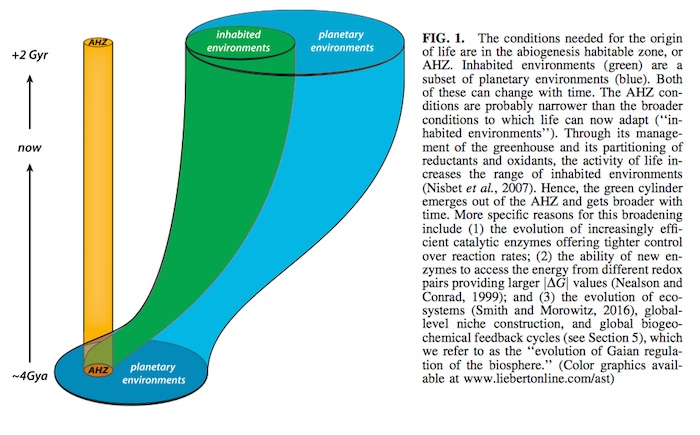
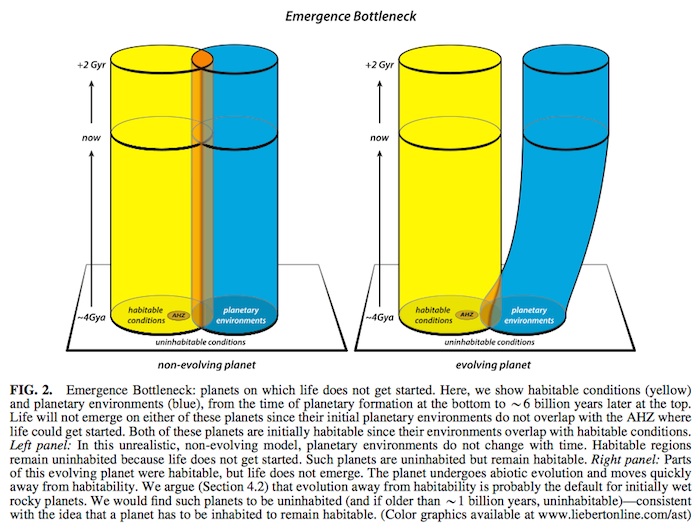
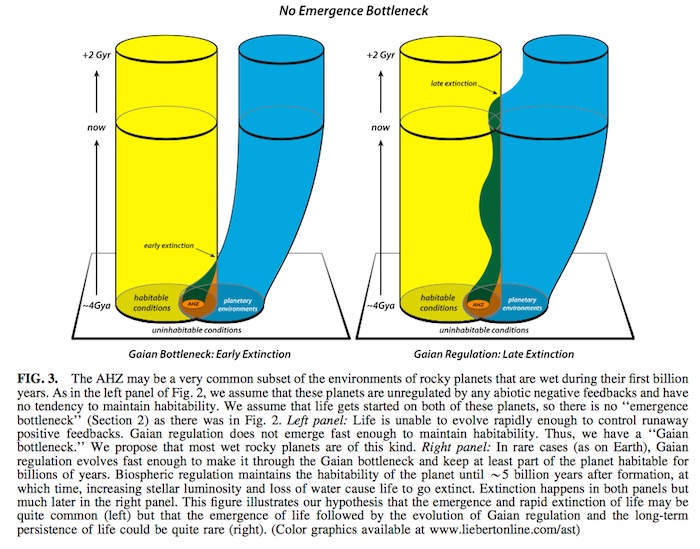
4429 Views