.
COMETWATCH 1 OCTOBER
Today's CometWatch entry is an image from Rosetta’s NAVCAM taken on 1 October 2015, at a distance of 1323 km from the nucleus of Comet 67P/Churyumov-Gerasimenko.
.
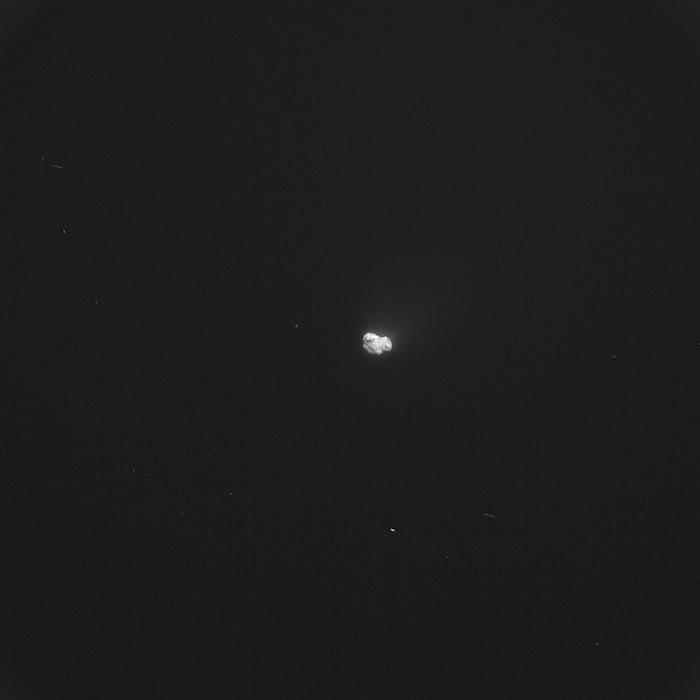
Single frame enhanced NAVCAM image of Comet 67P/C-G taken on 1 October 2015. Credits: ESA/Rosetta/NAVCAM – CC BY-SA IGO 3.0
-
The scale is 113 m/pixel and the image measures 115 km across. The contrast was increased to bring out the comet's activity.
From this perspective, the double-lobed shape of the nucleus is not as obvious as it is in some of the more iconic images of 67P/C-G; in this view, the large lobe is in the foreground, on the left, while the small lobe can be seen in the background, towards the right.
The Imhotep region dominates the view on the large lobe. To its right, parts of the newly identified regions on the comet's southern hemisphere are also visible: Anhur and Khonsu on the large lobe, Sobek on the neck and Wosret on the small lobe.
When this image was taken, Rosetta was approaching the nucleus again after having reached a distance of about 1500 km as part of a far excursion to study the comet's environment at large. The spacecraft is now about 600 km from the comet.
---
INTERPRETING IMAGES – MORE ON HOW THE COMET GOT ITS SHAPE
On 28 September, a scientific paper was published in Nature, presenting a view on the formation scenario of Comet 67P/Churyumov-Gerasimenko, based on Rosetta OSIRIS images (read our news report here). The paper, led by Dr Matteo Massironi of the University of Padova, Italy, evaluated two possible models to explain the comet's curious shape: the merging of two cometesimals or the erosion of a single object. Observational data and thorough analysis of the comet's gravity field pointed towards the first of the two hypotheses: 67P/C-G seems to have originated from two separately formed comets that merged at low speed.
The topic has generated a lot of discussion in the comments thread of this blog, in particular by proponents of an alternative explanation put forward by our blog readers Marco and A. Cooper who suggest that the comet was once a single body that has since been stretched into two separate lobes.
We asked Matteo to share his opinion not only on this subject but also on the general topic of how planetary images are interpreted in order to arrive at a robust scientific theory.
Here is what he wrote:
Sorry for my late reply, but I wanted some time to go through the blog where the stretching hypothesis is described before saying something about the supporting geological proofs. As a scientist, I do not normally read works that have not been rigorously reviewed and published in scientific journals. Probably that is my fault and I want to thank ESA to have created this interesting blog allowing so many people to be an active part of Rosetta’s discoveries.
As a geologist, I would first like to point out some basic principles to follow when dealing with space images for geological interpretations. I believe these suggestions could be of some help to anyone who is going to submit his or her own work on cometary geology and structure.
These principles can be synthetized as follows:
Be a good field geologist
Be rigorous
Never fall in love with theories (particularly your own ones)
Be short
1) Be a good field geologist
Whenever we observe a geological landscape we filter it on the basis of our own experience. Becoming a good field geologist is a hard task because it requires the combination of a deep knowledge of geological processes and a large amount of direct experience. I normally spend half of my time working on Earth geology and doing science based on field geology. Indeed, working in the field deeply increases your skills in correctly recognising geological features (tectonic, volcanic, glacial etc.). If you are not able to recognize different geological features you might fall into common perceptive traps by, for example, perceiving continuous illusory lines linking different unrelated geological objects.
You might like to enhance your own geological experience by stepping back from your laptop or work-station, leave 67P/C-G images where they are, wear a good couple of boots, have a walk and reach a panoramic view where rocks are beautifully exposed. Since Comet 67P/C-G displays layered material, you should choose a good exposure of a well-stratified sedimentary succession or a heavily foliated metamorphic sequence. Once you have reached your observational point, sit down, take a breath and start drawing what you see.
You should at this point be able to recognise different features: the primary ones, which are strata and foliations, the secondary ones, that can be faults and fractures, and the morphology which in your sketch is the line separating the sky from the landscape. Most probably you will see that the morphology might in places follow the primary structures, in other parts the secondary structures and in many others it simply follows his own shape given by erosional processes. Any matching exercise in geology should take into account only primary and secondary structures, while the morphological ones, if not related to the previous ones, should be avoided.
For example, restoring a faulted and sheared geological section should be based in collimating the primary structure after having recognised the faults where shear took place. The surface morphology should be avoided because it is affected by later erosional processes, which in any case on Earth are often much less relevant with respect to the ones happening on comets.
In substance, we cannot rely on the morphology of a heavily changing body such as a comet, but only on the expressions of its internal structural features.
In any case, after your panoramic drawing is completed, please walk into your panorama and see if what you have interpreted is correct (it would be better to be accompanied by a field geologist). After this simple exercise, come back to your work-station and try again to interpret the comet images from Rosetta. You will probably find something different than what you first thought.
2) Be rigorous
Any time I work on planetary images, I need correctly scaled and calibrated data that can be overlapped onto Digital Elevation Models or Shape models. In this way, I know perfectly what the possible errors are on my geo-structural interpretation. The Rosetta-OSIRIS team is really smart on these procedures and many people from MPI, DLR and LAM are involved in image calibration and shape model realisation (applying different methodologies). Hence, for example, I know that the error in the estimate of angular deviation from perpendicular between the strata and the local gravity vector is on average of 2° and in the worst case of 4°. In any case, my point is that any promising observation deserves a thorough and detailed investigation to understand the possible errors.
My advice “be rigorous” also means to go deeply through any published works before critically judging it.
3) Never fall in love with theories (particularly your own)
In general, science works from observations to hypotheses to models to theories. An observation may trigger a hypothesis, which should be tested with different approaches trying to find evidences that might undermine your own hypothesis.
For example, neck-enhanced erosion and simple contact binary of two young cometisimals were the two favoured scenarios for Comet 67P/C-G’s formation because they do not present any problems to existing theories related to Solar System formation and evolution. On the contrary, a contact binary of two fully-formed comets with an ordered onion-like inner structure raises relevant issues with pre-existing models (already correctly highlighted by some readers of this blog in the comment section).
In my opinion, these issues can be synthesized as follows: i) understanding how strata can form in the primordial Solar System, ii) how a comet might avoid any catastrophic collision during their lives, and iii) how low collisional impacts can happen in the primordial proto-planetary disk. The only issue that was already solved is the formation and stitching of contact binaries through low velocity collisions (see the publication The shape and structure of cometary nuclei as a result of low-velocity accretion by Jutzi & Asphaug for more information).
Due to the controversial implications that the onion-like contact binary raises, we tried to find other lines of evidence that might undermine what was apparent from the former observations. This is why, from the best fitting planes, we passed to the geological sections and afterwards worked on the angular relationships between strata and the local gravity vectors. All these independent observations based on primary structures support the view in which the comet derives from a contact binary of two comets with an onion-like interior.
Our cometary mission is called Rosetta because it has the potential to decipher the origin and evolution of the Solar System. We should put aside our previous theories and models and try to study images without forcing our observations to a particular model or another. Theories and new models will come later (stay tuned!).
4) Be short
When presenting your theories it is important to be short and concise – more words do not necessarily make a scientific hypothesis stronger.
With those points in mind, and after spending some time familiarising myself with the stretch hypothesis of Mr Cooper, and with the comments posted on the original thread, I have the following remarks:
-It seems that most of Mr Cooper’s observations are based on surface morphology with many primary and secondary features not correctly recognized (see point 1 above for advice on geological interpretation)
-He begins explaining the stretching hypothesis, providing the observations that should support his theory only afterwards. In general, science works the other way around, from observations to hypotheses to models to theories (see point 3 above). On the contrary, his observations in support of the stretching hypothesis are only based on putative matching points recognised on the surface morphology. Something else is really needed to corroborate the hypothesis.
-Some other blog readers and commenters have asserted that matching is enough and probably the same was for Alfred Wegener’s description of continental drifting and the later plate tectonics theories. In fact, this is not correct: even Wegener provided many independent lines of evidence including geodetic, geologic, geophysics, paleontological and paleo-climatic arguments. And the same was for plate tectonic, with additional paleo-magnetic and geostructural evidences.
- Relevant suggestions from the general public are valuable to scientists, but please take your time to become familiar with the supporting scientific literature before immediately arriving at a different conclusion.
- If anyone wants to submit a scientific paper on the stretching hypothesis (or any other topic!) please keep in mind the points above about geology, rigour and providing convincing evidence in a concise way.
Best wishes,
Matteo
---
COMETWATCH 9 OCTOBER
This image of Comet 67P/Churyumov-Gerasimenko was taken by Rosetta’s NAVCAM on 9 October from a distance of 579 km.
The image scale is 49.4 m/pixel and the image measures 50.5 km across. The contrast is increased to better see the comet’s activity, with several distinct active regions clearly visible.
.
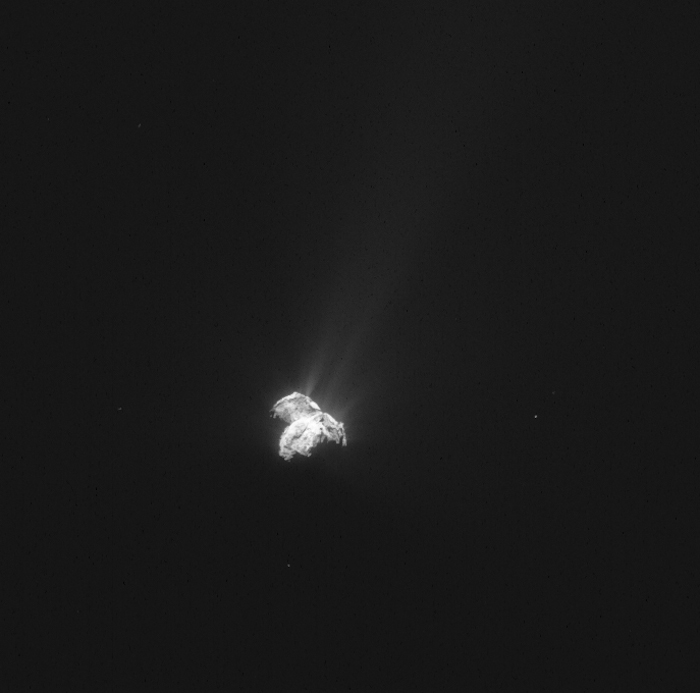
Single frame enhanced NAVCAM image of Comet 67P/C-G taken on 9 October 2015. Credits: ESA/Rosetta/NAVCAM – CC BY-SA IGO 3.0
-
In this orientation the comet’s small lobe is in the background, with primarily the Anuket region visible, and the large lobe is in the foreground with parts of Atum, Anubis and Khonsu visible.
Rosetta is currently on its return leg of a 1500 km far excursion to study the wider coma and plasma environment from afar, and is scheduled to reach a distance of 450 km from the comet by tomorrow (Saturday).
.
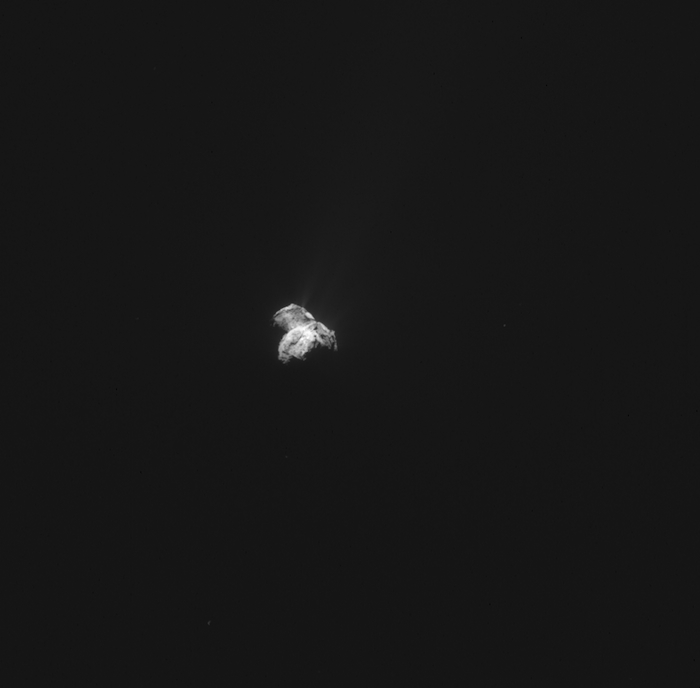
---
COMET JET IN 3D
OSIRIS scientists have created a spectacular anaglyph view of a jet seen blasting from the nucleus of Comet 67P/Churyumov-Gerasimenko in August.
Rosetta observed increased levels of cometary activity in the weeks around perihelion as the comet made its closest approach to the Sun along its orbit, at a distance of about 186 million km – between the orbits of Earth and Mars. On the approach, the increasing solar radiation heated up the comet’s nucleus, causing its frozen ices to escape as gas and stream out into space at an ever-greater rate, dragging the comet’s dust with it.
A period of peak of activity, where distinct jets increase in number and intensity, is thus expected in the weeks around perihelion. The jet shown here is one such example of the high level of activity seen over the last months; it was witnessed on 12 August, just one day before perihelion.
.
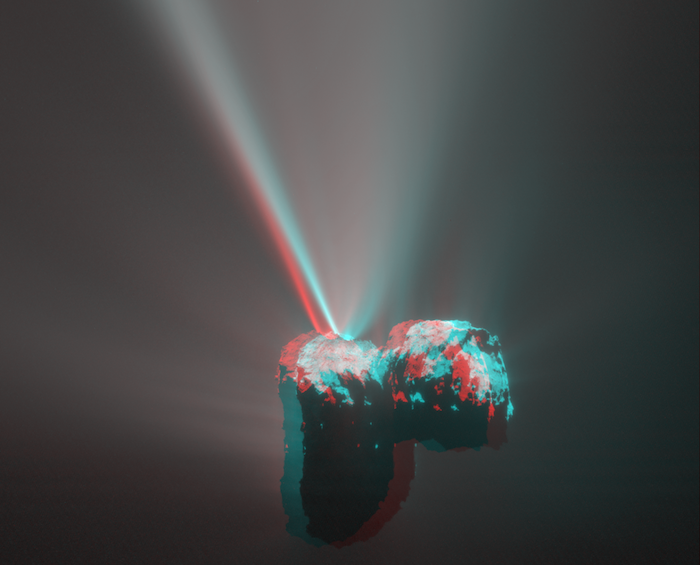
A 3D anaglyph view of Comet 67P/Churyumov-Gerasimenko based on two images acquired by Rosetta’s OSIRIS narrow angle camera on 12 August 2015, capturing a spectacular jet event. The two images are separated by 2 minutes 28 seconds, which corresponds to a stereo angle of 1.2 degrees. The image scale is 3.9 metres per pixel. In this orientation the Babi and Aker regions are visible on the large lobe to the left, while Ma’at and the circular Hatmehit depression are seen on the small lobe to the right. Diffuse dust emission and other jets are visible all around the nucleus. Acknowledgement: D. Romeuf (University Claude Bernard Lyon 1, France; images: ESA/Rosetta/MPS for OSIRIS Team MPS/UPD/LAM/IAA/SSO/INTA/UPM/DASP/IDA
.
But creating a 3d anaglyph of dynamic events like this is notoriously difficult: often the jets are too faint or their duration is too short to find two high-quality images taken several minutes apart that are suitable to pair together to create this type of view. However, the OSIRIS team got lucky with this particular event, capturing two images separated by about two-and-a-half minutes.
The image shows a bright, collimated jet embedded in a broader emission structure. The three dimensional perspective also reveals the conical shape of the jet and that the collimated feature is emitted towards the observer.
Jets have been seen to originate from a large diversity of morphological features on the comet’s surface, such as pits, cliffs or icy boulder fields. The physical processes responsible for the different jet structures are subject to much discussion, but anaglyphs like these can certainly help in gaining an understanding of their three dimensional form and evolution.
The image is best enjoyed with red-blue/green ‘3D’ anaglyph glasses. The left- and right-eye views have been extracted and are provided below.
.
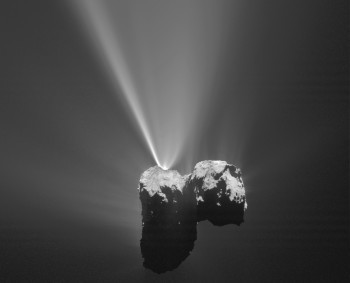
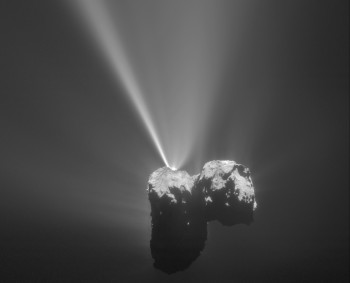
Quelle: ESA
-
Update: 24.10.2015
.
COMETWATCH 18 OCTOBER
Today's CometWatch entry was taken by Rosetta’s NAVCAM on 18 October 2015, at 433 km from the nucleus of Comet 67P/Churyumov-Gerasimenko.
.
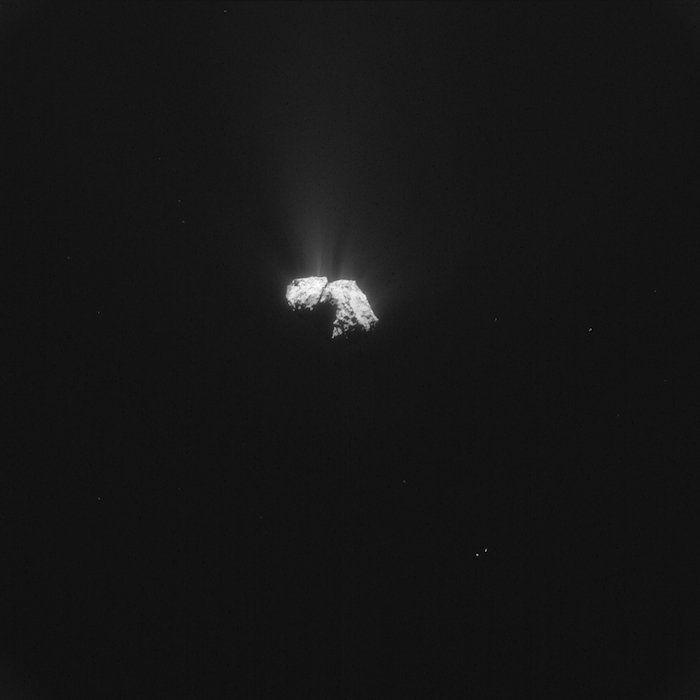
Single frame enhanced NAVCAM image of Comet 67P/C-G taken on 18 October 2015. Credits: ESA/Rosetta/NAVCAM – CC BY-SA IGO 3.0
-
The scale is 36.9 m/pixel and the image measures 37.8 km across. The contrast was increased to reveal the comet's activity, showing the glow of outflowing material from various regions on the nucleus.
In this view, the comet is oriented with the small lobe on the left and the large lobe on the right. Visible on the small lobe are parts of the Hatmehit, Maftet and Ma'at regions and, towards the centre of the image, the small regions of Nut and Serqet. Hints of the Anuket and Hapi regions can be seen on the comet's neck, while the large lobe presents the circular features of Seth alongside the smooth terrains of Anubis and parts of the complex Atum region.
The original 1024 x 1024 image of today's CometWatch is provided below:
.
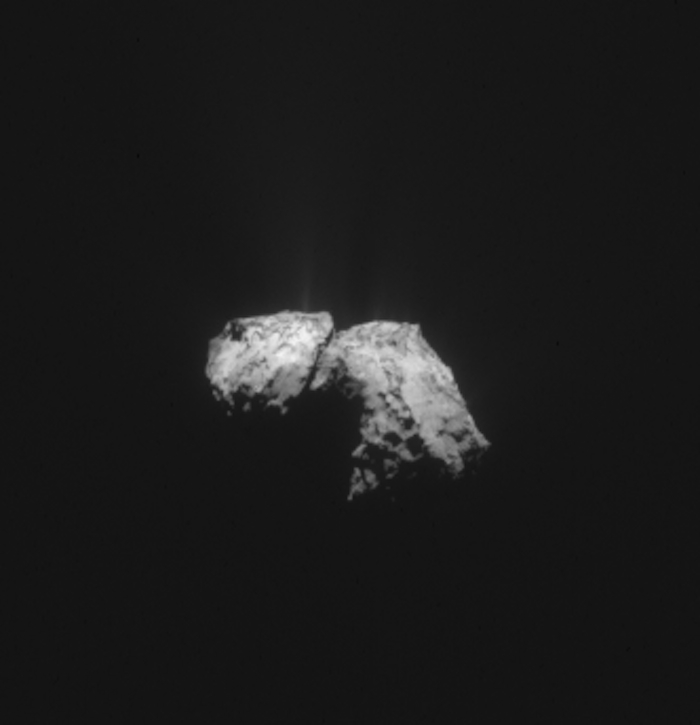
Quelle: ESA
-
Update: 29.10.2015
.
Komet Tschuri haut noch einen raus
Weltraumforscher sind verblüfft: Das Team der Rosetta-Mission hat in der Atmosphäre von Tschuri molekularen Sauerstoff gefunden. Der hätte da gar nicht sein dürfen.
Was hat die europäische Rosetta-Mission nicht schon an Nerven gekostet: Erst reiste die Sonde jahrelang durch das All, um den Kometen Tschurjumow-Gerassimenko zu treffen, dann landete Mini-Labor Philae vor rund einem Jahr knapp – ganz knapp – endlich auf ebenjenem "Tschuri", nur um anschließend in eine Art Winterschlaf zu verfallen und bloß gelegentlich daraus zu erwachen. Aber: Irgendwie läuft's. Zumindest wenn man berücksichtigt, dass das Rosetta-Team in den vergangenen Monaten doch immer wieder auf Interessantes stieß, das sich in Fachpublikationen gießen ließ.
Nun gibt es wieder Neuigkeiten vom Esa-Vorzeigeprojekt. Forscher haben erstmals Sauerstoffmoleküle (O2) in Tschuris Atmosphäre und damit auf einem Kometen überhaupt nachgewiesen. Das Sauerstoffgas müsse sehr alt sein und aus der Entstehungszeit des Sonnensystems stammen, schreiben die Wissenschaftler um André Bieler von der Universität Bern in ihrer aktuellen Studie (Nature, 2015). Die unerwartete Entdeckung stelle damit bisherige Modelle von der Entstehung unseres Systems infrage.
Stabil, aber einsam
Die Entdeckung gelang mit dem Massenspektrometer Rosina, das Rosetta mit sich durch das All trägt. Dank des Geräts konnten Forscher die chemische Zusammensetzung der Gaswolke untersuchen, die sich um den tauenden Kometen während seines Anflugs auf die Sonne zwischen September und März bildete. Erstmals ist das vor Ort gelungen, bisherige Messungen waren weit unpräziser, fanden nur aus großer Entfernung statt und mussten innerhalb weniger Minuten stattfinden. Das Rosetta-Team war also überzeugt, Neues zu finden. Was, ist dann aber doch überraschend: Molekularer Sauerstoff (O2) ist mit einem Anteil von 3,8 Prozent das vierthäufigste Gas in der Kometenatmosphäre. Häufiger gibt es bloß Wasser (H2O), Kohlenmonoxid (CO) und Kohlendioxid (CO2).
Quelle: Zeit
---
FIRST DETECTION OF MOLECULAR OXYGEN AT A COMET
ESA’s Rosetta spacecraft has made the first in situ detection of oxygen molecules outgassing from a comet, a surprising observation that suggests they were incorporated into the comet during its formation. This news story is mirrored from the main ESA web portal.
.
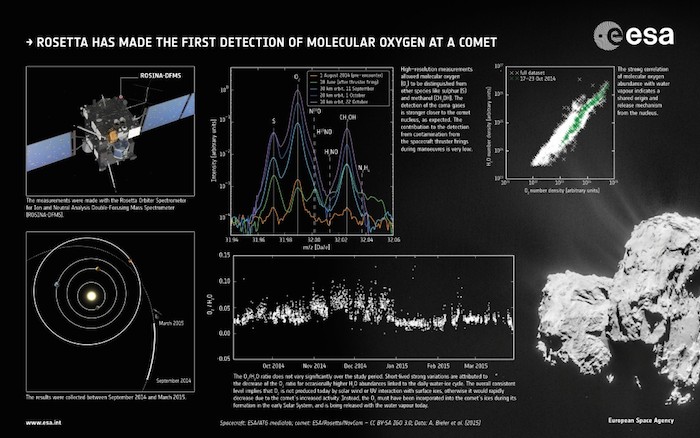
Rosetta has been studying Comet 67P/Churyumov–Gerasimenko for over a year and has detected an abundance of different gases pouring from its nucleus. Water vapour, carbon monoxide and carbon dioxide are the most prolific, with a rich array of other nitrogen-, sulphur- and carbon-bearing species, and even ‘noble gases’ also recorded.
Oxygen is the third most abundant element in the Universe, but the simplest molecular version of the gas, O2, has proven surprisingly hard to track down, even in star-forming clouds, because it is highly reactive and readily breaks apart to bind with other atoms and molecules.
For example, oxygen atoms can combine with hydrogen atoms on cold dust grains to form water, or a free oxygen split from O2 by ultraviolet radiation can recombine with an O2 molecule to form ozone (O3).
Despite its detection on the icy moons of Jupiter and Saturn, O2 had been missing in the inventory of volatile species associated with comets until now.
“We weren’t really expecting to detect O2 at the comet – and in such high abundance – because it is so chemically reactive, so it was quite a surprise,” says Kathrin Altwegg of the University of Bern, and principal investigator of the Rosetta Orbiter Spectrometer for Ion and Neutral Analysis instrument, ROSINA.
“It’s also unanticipated because there aren’t very many examples of the detection of interstellar O2. And thus, even though it must have been incorporated into the comet during its formation, this is not so easily explained by current Solar System formation models.”
.
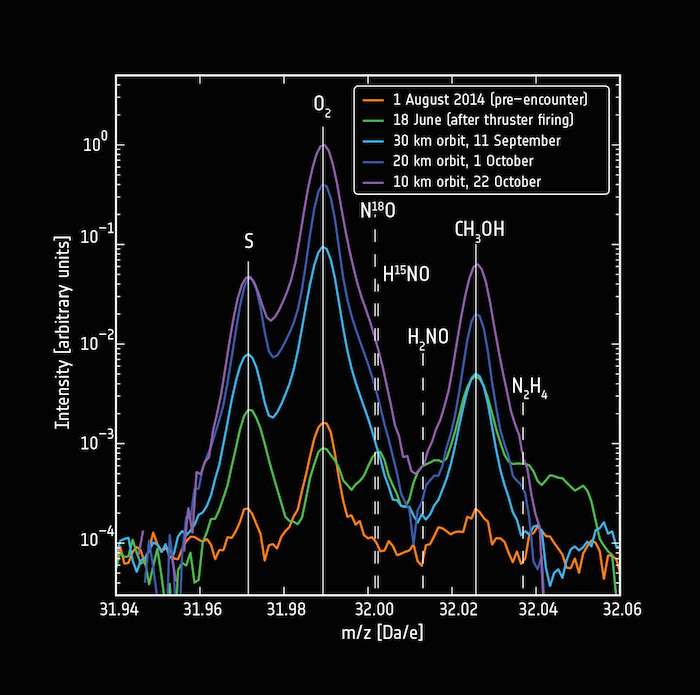
High-resolution measurements allowed molecular oxygen (O2) to be distinguished from other species like sulphur (S) and methanol (CH3OH). The detection of the coma gases is stronger closer to the comet nucleus, as expected. The contribution to the detection from contamination from the spacecraft thruster firings during manoeuvres is very low. Data from A. Bieler et al. (2015)
.
The team analysed more than 3000 samples collected around the comet between September 2014 and March 2015 to identify the O2. They determined an abundance of 1–10% relative to H2O, with an average value of 3.80 ± 0.85%, an order of magnitude higher than predicted by models describing the chemistry in molecular clouds.
The amount of molecular oxygen detected showed a strong relationship to the amount of water measured at any given time, suggesting that their origin on the nucleus and release mechanism are linked. By contrast, the amount of O2 seen was poorly correlated with carbon monoxide and molecular nitrogen, even though they have a similar volatility to O2. In addition, no ozone was detected.
Over the six-month study period, Rosetta was inbound towards the Sun along its orbit, and orbiting as close as 10–30 km from the nucleus. Despite the decreasing distance to the Sun, the O2/H2O ratio remained constant over time, and it also did not change with Rosetta’s longitude or latitude over the comet.
In more detail, the O2/H2O ratio was seen to decrease for high H2O abundances, an observation that might be influenced by surface water ice produced in the observed daily sublimation–condensation process.
The team explored the possibilities to explain the presence and consistently high abundance of O2 and its relationship to water, as well as the lack of ozone, by first considering photolysis and radiolysis of water ice over a range of timescales.
.
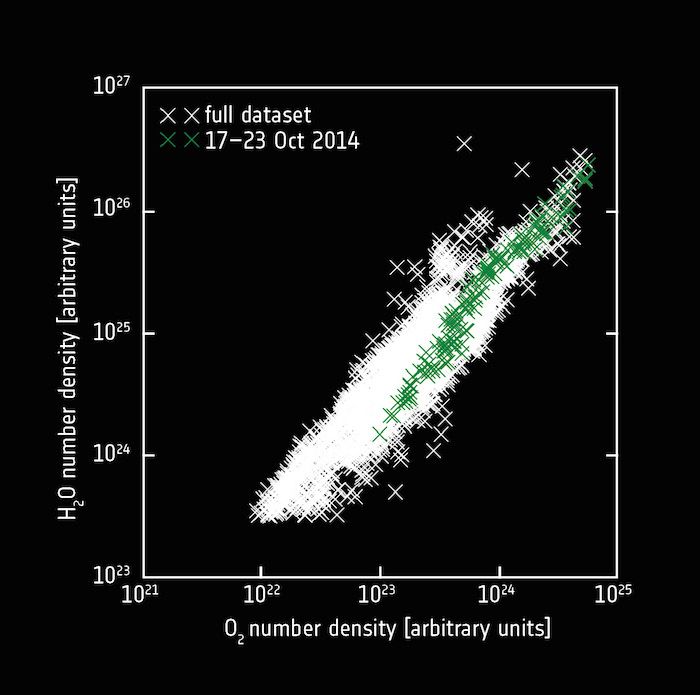
The strong correlation of molecular oxygen abundance with water vapour indicates a shared origin and release mechanism from the nucleus. Data from A. Bieler et al. (2015)
.
n photolysis, photons break bonds between molecules, whereas radiolysis involves more energetic photons or fast electrons and ions depositing energy into ice and ionising molecules – a process observed on icy moons in the outer Solar System, and in Saturn’s rings. Either process can, in principle, lead to the formation and liberation of molecular oxygen.
Radiolysis will have operated over the billions of years that the comet spent in the Kuiper Belt and led to the build-up of O2 to a few metres depth. But these top layers must all have been removed in the time since the comet moved into its inner Solar System orbit, ruling this out as the source of the O2 seen today.
More recent generation of O2 via radiolysis and photolysis by solar wind particles and UV photons should only have occurred in the top few micrometres of the comet.
.
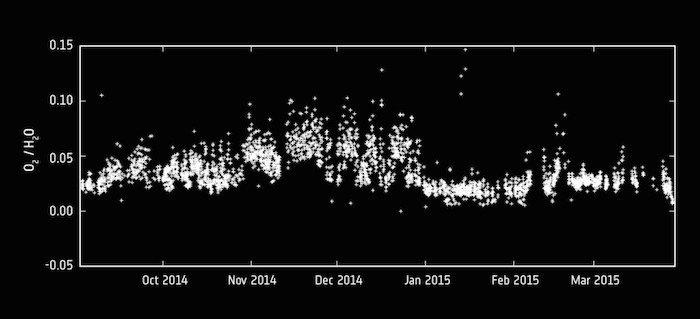
The O2/H2O ratio does not vary significantly over the study period. Short-lived strong variations are attributed to the decrease of the O2 ratio for occasionally higher H2O abundances linked to the daily water-ice cycle. The overall consistent level implies that O2 is not produced today by solar wind or UV interaction with surface ices, otherwise it would rapidly decrease due to the comet's increased activity. Instead the O2 must have been incorporated into the comet's ices during its formation in the early Solar System, and is being released with the water vapour today. Data by A. Bieler et al. (2015)
-
“But if this was the primary source of the O2 then we would have expected to see a decrease in the O2/H2O ratio as this layer was removed during the six-month timespan of our observations,” says Andre Bieler of the University of Michigan and lead author of the paper describing the new results in the journal Nature this week.
“The instantaneous generation of O2 also seems unlikely, as that should lead to variable O2 ratios under different illumination conditions. Instead, it seems more likely that primordial O2 was somehow incorporated into the comet’s ices during its formation, and is being released with the water vapour today.”
In one scenario, gaseous O2 would first be incorporated into water ice in the early protosolar nebula stage of our Solar System. Chemical models of protoplanetary discs predict that high abundances of gaseous O2 could be available in the comet forming zone, but rapid cooling from temperatures above –173ºC to less than –243ºC would be required to form water ice with O2 trapped on dust grains. The grains would then have to be incorporated into the comet without being chemically altered.
“Other possibilities include the Solar System being formed in an unusually warm part of a dense molecular cloud, at temperatures of 10–20ºC above the –263ºC or so typically expected for such clouds,” says Ewine van Dishoeck of Leiden Observatory in the Netherlands, co-author of the paper.
“This is still consistent with estimates for the comet formation conditions in the outer solar nebula, and also with previous findings at Rosetta’s comet regarding the low abundance of N2.”
Alternatively, radiolysis of icy dust grains could have taken place prior to the comet’s accretion into a larger body. In this case, the O2 would remain trapped in the voids of the water ice on the grains while the hydrogen diffused out, preventing the reformation of O2 to water, and resulting in an increased and stable level of O2 in the solid ice.
Incorporation of such icy grains into the nucleus could explain the observed strong correlation with H2O observed at the comet today.
“Regardless of how it was made, the O2 was also somehow protected during the accretion stage of the comet: this must have happened gently to avoid the O2 being destroyed by further chemical reactions,” adds Kathrin.
“This is an intriguing result for studies both within and beyond the comet community, with possible implications for our models of Solar System evolution,” says Matt Taylor, ESA’s Rosetta project scientist.
Quelle: ESA
4978 Views