.
Is the International Space Station the last aluminum spacecraft?
Robert T. Bigelow has developed the inflatable Bigelow Expanded Activity Module, an aluminum habitation, to test in space. Pictured is a one-third scale model. (NASA)
.
Late this year or early next, NASA will build an addition to the International Space Station, increasing the orbital laboratory’s size from eight rooms to nine. The new room is like no other on the station, and will be very easy to construct: Just connect to a docking port, fill with compressed air, and voilà! Instant space habitat.
The hard part was the 15 years of research and development that Bigelow Aerospace in North Las Vegas needed to create the Bigelow Expandable Activity Module, or BEAM. Initially scheduled for a September launch, BEAM’s test deployment is now delayed due to the post-launch explosion of a SpaceX Falcon 9 rocket bound for the ISS on June 28 — and no one yet knows how long that delay will be. Once BEAM does reach its destination, it will undergo two years of intensive testing, a trial run for a technology that could play a significant role in future human spaceflight and low-Earth-orbit commercial ventures: inflatable spacecraft.
Bigelow’s inflatable is, in a sense, the resurrection of a canceled NASA program. In the 1990s, NASA developed TransHab, or Transit Habitat, an inflatable living area to test in space with the goal of using such a container to transport humans to Mars and to replace the International Space Station’s aluminum habitation module. TransHab got only as far as ground testing before Congress cut the program’s funding in 2000. Real estate billionaire and space enthusiast Robert T. Bigelow purchased the rights to the patents that NASA filed for the technology.
Bigelow Aerospace picked up where TransHab left off, advancing research and development and eventually putting two inflatable test modules—Genesis I and II—into orbit in 2006 and 2007. Both modules, each the size of a van, remain in orbit today. Their batteries ran out years ago; eventually they’ll reenter the atmosphere and burn up. But they served their purpose. “Genesis I and II validated our basic architecture,” says Mike Gold, Bigelow’s Director of Washington, D.C. Operations & Business Growth. “From a technical perspective, these spacecraft showed that expandable systems could survive the rigors of launch, that our deployment process would work, and that we could successfully integrate windows into an expandable habitat structure.”
Inflatable habitats in space have advantages over conventional metal structures. First, they’re a lot cheaper to get into orbit. One reason is weight: BEAM, designed to expand to 16 cubic meters, or about the size of a 10- by 12-foot room, weighs only 3,000 pounds at launch. Its density—that is, its mass divided by its volume—is 88 kilograms per cubic meter. By comparison, the density of the U.S. lab at the International Space Station, Destiny, is 137 kilograms per cubic meter. The ISS’s Tranquility module has a density of 194 kilograms per cubic meter.
Inflatables are also appealingly compact. Folded into its launch configuration, BEAM takes up a space five feet by seven feet. Gold cites BEAM’s modest cost— 17.8 million—as one of its key advantages over older technologies: “I can’t think of any other substantial hardware that has been done, or almost any other project that’s been done, for such a relatively minor amount of money,” he says.
Reducing the size and weight of the payload at launch is what saves taxpayers money. “You gain tremendously in terms of launch efficiency, and that’s the hardest, most expensive thing about space—getting out of Earth’s gravity well,” says George Zamka, a former shuttle astronaut who worked for the FAA’s Office of Commercial Space Transportation before joining Bigelow Aerospace last year.
Rajib Dasgupta, BEAM project manager for NASA, says inflatables are one concept that the space agency is studying for habitation inside cislunar space—the sphere formed by the moon’s orbit of Earth. “Successful BEAM demonstration on ISS will certainly be a giant stepping stone to future deep-space exploration habitats,” he says.
Inflation Evaluation
BEAM was scheduled to be launched by SpaceX CRS-8, a cargo resupply mission to the ISS intially scheduled for September 2, though it will now be delayed as a result of the SpaceX explosion. (Bigelow’s Gold will only say he remains hopeful that BEAM will reach the station “this calendar year.”) Once BEAM arrives, it will face two years of engineering tests. But its first hurdle maybe be simply overcoming negative associations with the word “inflatable.”
“People sometimes have a bad perception of inflatable structures because of their experience with low-cost, poorly made products such as pool toys that leak, or party balloons that burst,” says David Cadogan, director of engineering for ILC Dover in Frederica, Delaware, a firm that has worked with NASA for decades, developing spacesuits, airbags for the Mars rovers, and airbags for Boeing’s proposed Crew Space Transportation-100 vehicle. But every day we entrust our lives to inflatable structures: car tires and air bags, emergency escape slides in airplanes, angioplasty surgeries.
Of course, inflatable habitats have never housed human beings in space before. NASA and its contractors have half a century’s worth of experience with aluminum pressure vessels; they know how to assemble them in space, how to inspect and maintain them, how to analyze their structural loads, and how to control fractures in them. They also know aluminum’s tolerance of—and vulnerability to—impacts from micrometeoroids and orbital debris. Engineers have developed ways to monitor impacts, find leaks, analyze damage, and even make limited repairs.
Steve Stich, director of exploration, integration and science at NASA’s Johnson Space Center, says inflatable habitats may someday be integrated with metal pressure vessels, but the agency needs to learn a lot more about how inflatables hold up against the hazards of space: radiation exposure, thermal cycling, debris impact. For example, BEAM has a metal structure at the end that berths to the ISS—it’s known as a common berthing mechanism. Loading forces from the station will place stresses on BEAM, particularly where the berthing mechanism attaches to the station, and also where the berthing mechanism attaches to BEAM’s fabric shell.
No one yet knows whether inflatable habitats can safely dock to other spacecraft, and whether an airlock can be integrated into an inflatable habitat. Stich believes that for high-stress applications like docking, aluminum will likely remain: “I don’t see us totally ever phasing out metallic structures,” he says.
One challenge, Stich adds, is how to develop inflatables that can be outfitted with life support, crew quarters, and other systems prior to launch; if not, astronauts will have to set those up once the habitat is deployed in space. Conventional modules at the space station typically arrive with equipment already integrated into the structure.
George Studor, a retired NASA senior project engineer who now consults, through various contractors, for the NASA Engineering and Safety Center, says inflatable habitats face an uphill battle to win the kind of confidence NASA has in the metal ships it has been building for half a century.
“It takes heritage to have confidence in a technology,” Studor says. “Even if the inflatable Bigelow space station turns out really great, it doesn’t mean that there aren’t faults with that thing…. There haven’t been enough of them made. There hasn’t been enough materials experience and testing. It becomes a more risky space venture than what we would normally do. But because of its potential, NASA has been working with Bigelow for many years to help the technology mature.”
An Idea Nearly as Old as NASA
NASA first began studying the possibilities of inflatable structures around 1960, when researchers at NASA’s Langley Research Center in Virginia drew up plans for a doughnut-shaped space station. In another inflatables project, known as Echo, NASA launched giant Mylar-coated balloons into orbit in 1960 and 1964 and bounced radio signals off them. In 1965, the agency developed concepts for inflatable moon habitats, and in 1967 it studied the idea of an air-filled space station nicknamed Moby Dick, apparently due to its large dimensions.
TransHab emerged 30 years later as a project at NASA’s Johnson Space Center. The effort was led by William Schneider, who had worked on micrometeoroid protection for the space shuttle. Schneider had already retired when TransHab was canceled in 2000, but he has consulted with Bigelow Aerospace.
TransHab faced skepticism from the start. NASA’s Kriss Kennedy, a space architect who helped create the inflatable and coined the name, recalled in Air & Space (“Launch. Inflate. Insert Crew,” May, 1999) that during public talks he would pop a balloon to drive the point home that this is a balloon; inflatable structures are not. During the short-lived TransHab program, NASA engineers developed inflatable habitats with a foot-thick, 16-layer shell of foam and fabric that stood up to ballistics tests designed to simulate strikes by micrometeoroids and orbital debris.
The actual architecture of TransHab included three thin-film air bladders covered by alternating layers of ceramic fabric, polyurethane foam, and Kevlar. The ceramic fabric, called Nextel, was sandwiched by three-inch layers of foam.Together, the layers served to protect against micrometeoroids. The Kevlar webbing made up TransHab’s pressure-holding restraint layer, which was woven like a rug to reduce the number of seams and maximize strength. Inside TransHab, two-inch-thick walls surrounding bedrooms would be filled with water to shield crew members from radiation.
BEAM represents a generation of refinement to that earlier design. From inside to outside, says Dasgupta, it includes a bladder, restraint system, micrometeoroid/orbital debris protection, insulation, and an external thermal blanket. (BEAM’s precise makeup is proprietary.) Gold says BEAM’s “Kevlar-like” protective layers will measure up. “We have done side-by-side hyper-velocity impact testing with portions of the ISS’s [micrometeoroid/orbital debris protection] layers,” he says. “Our system offers equal if not superior protections to what’s on the ISS today.”
He pauses before choosing a dramatic example. “If you’re about to get shot, would you rather have aluminum in front of you or a Kevlar vest?”
Trial in Space
Once SpaceX’s uncrewed Dragon cargo spacecraft reaches the ISS, the station’s robotic arm will be used to attach BEAM to the aft section of the Node 3 module. With the hatch to the station closed, air tanks inside BEAM will pressurize the module. Inside, a telescoping structure will expand as BEAM inflates. Made of an aluminum alloy, the structure is designed to provide rigidity in case a micrometeoroid or piece of orbital debris penetrates the habitat, says Dasgupta.
The primary performance requirement for BEAM is to demonstrate that it can be launched, deploy on the ISS, inflate, and maintain long-term pressure without leakage. Another key objective is to determine how well an inflatable structure in low Earth orbit can protect astronauts from radiation. BEAM will be outfitted with radiation sensors, and data from them will be compared to corresponding data collected on the ISS aluminum modules. Solar flares pose an additional radiation risk.
Gold says BEAM should offer better radiation protection than metal: When metallic structures absorb radiation, the shielding material can itself emit “secondary radiation.” When high-energy particles smash into atoms in a spacecraft’s metallic shielding, the collisions produce a shower of nuclear byproducts—neutrons and other particles—that then enter the spacecraft. Secondary radiation can be more dangerous than the original radiation from space. “The non-metallic structure of the BEAM substantially reduces the secondary radiation effect that otherwise occurs within metallic structures,” Gold adds.
Once you’re beyond low Earth orbit and exposure to cosmic radiation increases, neither metallic nor fabric construction can fully protect astronauts—a longer-term concern as future astronauts travel to the moon, Mars, and beyond. “The only thing you could do there is provide a very massive dense material to absorb it, basically,” Zamka acknowledges. “It’s parts of atoms coming at you.”
Apart from the need to protect astronauts, the greatest engineering challenge for BEAM is likely maintaining structural integrity over time—specifically, avoiding a phenomenon known as “creep rupture,” says ILC Dover’s Cadogan. Creep rupture occurs when the constant loading of materials at high percentages of their ultimate strength leads to an elongation of the material, and eventual failure.
However, if you can design and test a structure so loading is kept below 25 percent of the materials’ ultimate strength (for most structural materials), creep rupture shouldn’t be a problem. Although some materials are more susceptible to this type of stress than others, all materials have some degree of susceptibility, says Cadogan. Good engineering can mitigate the problem. One familiar example? Window glass. Two hundred years ago, glass would sag over time—an effect of gravity. Modern materials design has solved this vulnerability.
Cadogan says that BEAM’s manufacturing challenges are even more daunting than its engineering challenges. For example, ILC Dover welds polymer-coated fabrics to create bladders that retain inflation gas. These seals are made by applying heat and pressure to the materials in a highly controlled process. “Then there are the sewing operations that are used to create the restraint—the part that goes over the bladder and supports all the pressurization and structural loads,” Cadogan says. “Sewing also has parameters that require control, including thread tension, needle sharpness, stitches per inch, etc….You just have to set up the machines correctly, have proficient operators, and inspect and test everything well before flight.”
At the end of BEAM’s two-year mission, its last test will be when the station’s robotic arm successfully jettisons it from the ISS. The robotic jettison of a large, 3,000-pound structure from the station has never been attempted. Once detached, BEAM is expected to enter the atmosphere and burn up within a year.
Room to Move
Inflatables offer another clear benefit: more habitable space. BEAM is relatively small, but an operational module that Bigelow is developing, called B330, will offer 330 cubic meters of habitable volume. The International Space Station contains 916 cubic meters of pressurized volume—only about three times that of a single B330 module.
As a rule, astronauts enjoy about double the volume of a similar space on Earth, because in micro-gravity they have access to the entire area, from ceiling to floor, and in any orientation. The space station is a massive structure—with its extended solar arrays, about the size of a football field. But thinking about the ISS in that way can be deceiving. “Inside, you don’t get all that,” Zamka says. “It’s small and constrained by whatever node you happen to be in, whether it’s Tranquility or Serenity or Unity…. You’re in this kind of tube-like existence.”
Inflatable modules would offer astronauts more space. “I think they’ll notice that difference, particularly if they look at this expanded volume for traveling on long missions in deep space,” says Zamka.
The current plan calls for crew members to enter BEAM once every three months, although that may change, says Dasgupta. Their job will be to collect sensor data, perform surface sampling, change out radiation area monitors, and inspect the general condition of the module. BEAM’s ventilation is passive; it takes air pushed from the station through a duct. Air circulation inside BEAM will help prevent condensation. The module has no windows, though future designs could conceivably accommodate them.
“No hard time limit has been established for crew ingress, but since the ISS crew is busy all year round conducting ISS research, we would like to limit crew ingress to a few hours,” Dasgupta says.
NASA doesn’t plan to stow any equipment or hardware inside BEAM, and the module will have no internal power. Inside, crew members will carry battery-operated lights.
BEAM could become popular with astronauts, not only because of the extra space but also because it should be relatively quiet compared with other modules. Gold says, “We believe the BEAM could be an oasis.”
Assuming BEAM performs well, Bigelow Aerospace envisions B330 modules used as stand-alone space stations for the private sector. Pharmaceutical and materials science firms, for example, could use B330 modules as laboratories for product development, says Gold. (He declines to say how the B330 modules will be priced.) The B330s accommodate six, and Bigelow hopes they will become integral to deep-space missions—crashpads to keep astronauts from being confined to a capsule, like NASA’s planned Orion spacecraft.
“Obviously there is not sufficient volume [with Orion alone] for long-duration missions,” says Gold. However, if “you attach a habitat to a propulsion system and/or capsule, you’ve got a pretty robust system for beyond-LEO exploration to the moon, Mars and beyond.”
In this respect, NASA’s shelved TransHab program is truly on the verge of being reborn. Zamka says the B330 perfectly complements NASA’s Orion spacecraft. “[Orion] is a transfer vehicle. It’s supposed to transfer astronauts from Earth to another place,” he says. “We’re that other place.”
.
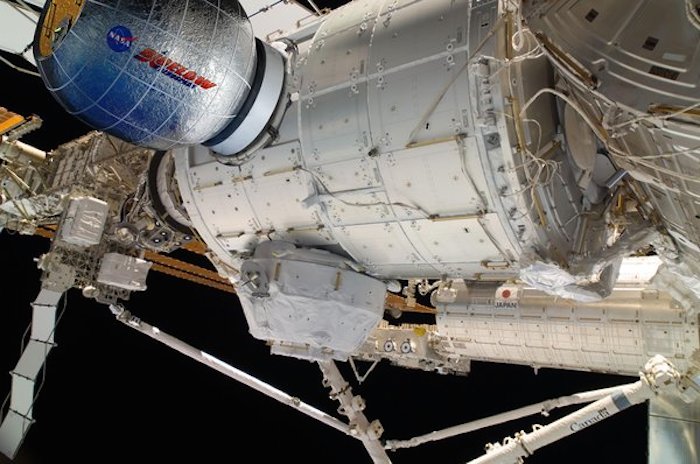
Concept illustration of BEAM docked to the ISS. Bigelow hopes the two-year test flight will demonstrate that space inflatables are versatile, economical, and safe. (Bigelow Aerospace)
Quelle: Air&Space
4039 Views