.
Hidden away in the secret depths of the Skunk Works, a Lockheed Martin research team has been working quietly on a nuclear energy concept they believe has the potential to meet, if not eventually decrease, the world’s insatiable demand for power.
Dubbed the compact fusion reactor (CFR), the device is conceptually safer, cleaner and more powerful than much larger, current nuclear systems that rely on fission, the process of splitting atoms to release energy. Crucially, by being “compact,” Lockheed believes its scalable concept will also be small and practical enough for applications ranging from interplanetary spacecraft and commercial ships to city power stations. It may even revive the concept of large, nuclear-powered aircraft that virtually never require refueling—ideas of which were largely abandoned more than 50 years ago because of the dangers and complexities involved with nuclear fission reactors.
.
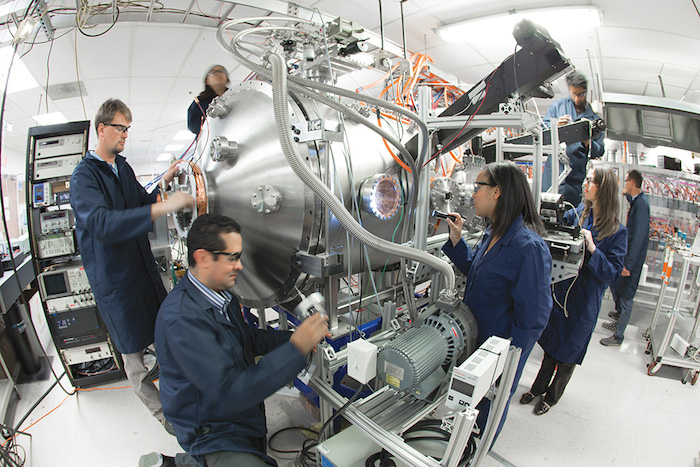
Yet the idea of nuclear fusion, in which atoms combine into more stable forms and release excess energy in the process, is not new. Ever since the 1920s, when it was postulated that fusion powers the stars, scientists have struggled to develop a truly practical means of harnessing this form of energy. Other research institutions, laboratories and companies around the world are also pursuing ideas for fusion power, but none have gone beyond the experimental stage. With just such a “Holy Grail” breakthrough seemingly within its grasp, and to help achieve a potentially paradigm-shifting development in global energy, Lockheed has made public its project with the aim of attracting partners, resources and additional researchers.
Although the company released limited information on the CFR in 2013, Lockheed is now providing new details of its invention. Aviation Week was given exclusive access to view the Skunk Works experiment, dubbed “T4,” first hand. Led by Thomas McGuire, an aeronautical engineer in the Skunk Work’s aptly named Revolutionary Technology Programs unit, the current experiments are focused on a containment vessel roughly the size of a business-jet engine. Connected to sensors, injectors, a turbopump to generate an internal vacuum and a huge array of batteries, the stainless steel container seems an unlikely first step toward solving a conundrum that has defeated generations of nuclear physicists—namely finding an effective way to control the fusion reaction.
“I studied this in graduate school where, under a NASA study, I was charged with how we could get to Mars quickly,” says McGuire, who earned his Ph.D. at the Massachusetts Institute of Technology. Scanning the literature for fusion-based space propulsion concepts proved disappointing. “That started me on the road and [in the early 2000s], I started looking at all the ideas that had been published. I basically took those ideas and melded them into something new by taking the problems in one and trying to replace them with the benefits of others. So we have evolved it here at Lockheed into something totally new, and that’s what we are testing,” he adds.
To understand the breakthroughs of the Lockheed concept, it is useful to know how fusion works and how methods for controlling the reaction have a fundamental impact on both the amount of energy produced and the scale of the reactor. Fusion fuel, made up of hydrogen isotopes deuterium and tritium, starts as a gas injected into an evacuated containment vessel. Energy is added, usually by radio-frequency heating, and the gas breaks into ions and electrons, forming plasma.
The superhot plasma is controlled by strong magnetic fields that prevent it from touching the sides of the vessel and, if the confinement is sufficiently constrained, the ions overcome their mutual repulsion, collide and fuse. The process creates helium-4, freeing neutrons that carry the released energy kinetically through the confining magnetic fields. These neutrons heat the reactor wall which, through conventional heat exchangers, can then be used to drive turbine generators.
Until now, the majority of fusion reactor systems have used a plasma control device called a tokamak, invented in the 1950s by physicists in the Soviet Union. The tokamak uses a magnetic field to hold the plasma in the shape of a torus, or ring, and maintains the reaction by inducing a current inside the plasma itself with a second set of electromagnets. The challenge with this approach is that the resulting energy generated is almost the same as the amount required to maintain the self-sustaining fusion reaction.
.
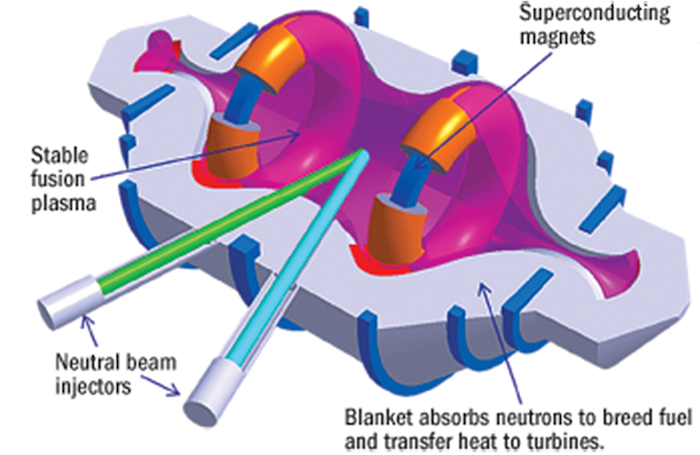
An advanced fusion reactor version, the International Thermonuclear Experimental Reactor (ITER), being built in Cadarache, France, is expected to generate 500 MW. However, plasma is not due to be generated until the late 2020s, and derivatives are not likely to be producing significant power until at least the 2040s.
The problem with tokamaks is that “they can only hold so much plasma, and we call that the beta limit,” McGuire says. Measured as the ratio of plasma pressure to the magnetic pressure, the beta limit of the average tokamak is low, or about “5% or so of the confining pressure,” he says. Comparing the torus to a bicycle tire, McGuire adds, “if they put too much in, eventually their confining tire will fail and burst—so to operate safely, they don’t go too close to that.” Aside from this inefficiency, the physics of the tokamak dictate huge dimensions and massive cost. The ITER, for example, will cost an estimated $50 billion and when complete will measure around 100 ft. high and weigh 23,000 tons.
The CFR will avoid these issues by tackling plasma confinement in a radically different way. Instead of constraining the plasma within tubular rings, a series of superconducting coils will generate a new magnetic-field geometry in which the plasma is held within the broader confines of the entire reaction chamber. Superconducting magnets within the coils will generate a magnetic field around the outer border of the chamber. “So for us, instead of a bike tire expanding into air, we have something more like a tube that expands into an ever-stronger wall,” McGuire says. The system is therefore regulated by a self-tuning feedback mechanism, whereby the farther out the plasma goes, the stronger the magnetic field pushes back to contain it. The CFR is expected to have a beta limit ratio of one. “We should be able to go to 100% or beyond,” he adds.
This crucial difference means that for the same size, the CFR generates more power than a tokamak by a factor of 10. This in turn means, for the same power output, the CFR can be 10 times smaller. The change in scale is a game-changer in terms of producibility and cost, explains McGuire. “It’s one of the reasons we think it is feasible for development and future economics,” he says. “Ten times smaller is the key. But on the physics side, it still has to work, and one of the reasons we think our physics will work is that we’ve been able to make an inherently stable configuration.” One of the main reasons for this stability is the positioning of the superconductor coils and shape of the magnetic field lines. “In our case, it is always in balance. So if you have less pressure, the plasma will be smaller and will always sit in this magnetic well,” he notes.
Overall, McGuire says the Lockheed design “takes the good parts of a lot of designs.” It includes the high-beta configuration, the use of magnetic field lines arranged into linear ring “cusps” to confine the plasma and “the engineering simplicity of an axisymmetric mirror,” he says. The “axisymmetric mirror” is created by positioning zones of high magnetic field near each end of the vessel so that they reflect a significant fraction of plasma particles escaping along the axis of the CFR. “We also have a recirculation that is very similar to a Polywell concept,” he adds, referring to another promising avenue of fusion power research. A Polywell fusion reactor uses electromagnets to generate a magnetic field that traps electrons, creating a negative voltage, which then attracts positive ions. The resulting acceleration of the ions toward the negative center results in a collision and fusion.
The team acknowledges that the project is in its earliest stages, and many key challenges remain before a viable prototype can be built. However, McGuire expects swift progress. The Skunk Works mind-set and “the pace that people work at here is ridiculously fast,” he says. “We would like to get to a prototype in five generations. If we can meet our plan of doing a design-build-test generation every year, that will put us at about five years, and we’ve already shown we can do that in the lab.” The prototype would demonstrate ignition conditions and the ability to run for upward of 10 sec. in a steady state after the injectors, which will be used to ignite the plasma, are turned off. “So it wouldn’t be at full power, like a working concept reactor, but basically just showing that all the physics works,” McGuire says.
.
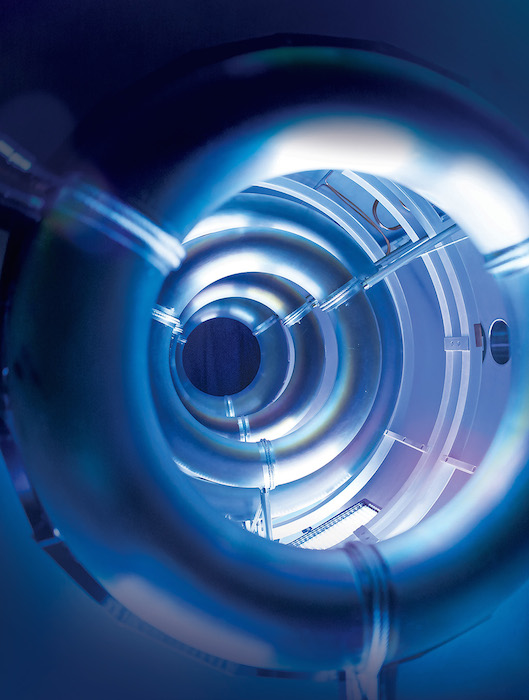
An initial production version could follow five years after that. “That will be a much bigger effort,” he says, suggesting that transition to full-scale manufacturing will necessarily involve materials and heat-transfer specialists as well as gas-turbine makers. The early reactors will be designed to generate around 100 MW and fit into transportable units measuring 23 X 43 ft. “That’s the size we are thinking of now. You could put it on a semi-trailer, similar to a small gas turbine, put it on a pad, hook it up and can be running in a few weeks,” McGuire says. The concept makes use of the existing power infrastructures to enable the CFR to be easily adapted into the current grid. The 100-MW unit would provide sufficient power for up to 80,000 homes in a power-hungry U.S. city and is also “enough to run a ship,” he notes.
Lockheed estimates that less than 25 kg (55 lb.) of fuel would be required to run an entire year of operations. The fuel itself is also plentiful. Deuterium is produced from sea water and is therefore considered unlimited, while tritium is “bred” from lithium. “We already mine enough lithium to supply a worldwide fleet of reactors, so with tritium you never have too much built up, and that’s what keeps it safe. Tritium would be a health risk if there were enough released, but it is safe enough in small quantities. You don’t need very much to run a reactor because it is a million times more powerful than a chemical reaction,” McGuire notes.
Although the first-generation reactors will have radioactive parts at the ends of their lives, such as some steel elements in the shell, McGuire says the contamination situation “is an order of magnitude better” than that of contemporary fission systems. “There is no long-lived radiation. Fission reactors’ stuff will be there forever, but with fusion materials, after 100 years then you are good.” Contamination levels for fusion will improve with additional materials research, he believes. “It’s been a chicken-and-egg situation. Until we’ve had a good working fusion system, there has not been money to go off and do the hard-core materials research,” McGuire says. “So we believe the first generation is good enough to go out and do, and then it will only improve in time.” Old CFR steel shell parts can be disposed of with “a shallow burial in the desert, similar to medical waste today. That’s a major difference to today’s fission systems.”
Operational benefits include no risks of suffering a meltdown. “There is a very minimal amount of radioactive tritium—it’s on the order of grams—so the potential release is very minimal. In addition, there is not enough to be a risk of proliferation. Tritium is used in nuclear weapons but in a much larger inventory than would be involved here, and that’s because you are continually making just enough to feed back in [to maintain the reaction],” he adds.
Preliminary simulations and experimental results “have been very promising and positive,” McGuire says. “The latest is a magnetized ion confinement experiment, and preliminary measurements show the behavior looks like it is working correctly. We are starting with the plasma confinement, and that’s where we are putting most of our effort. One of the reasons we are becoming more vocal with our project is that we are building up our team as we start to tackle the other big problems. We need help and we want other people involved. It’s a global enterprise, and we are happy to be leaders in it.”
Quelle: Aviation Week
4471 Views