.
The universe has undergone several dramatic changes in its 13.7 billion-year history, although our knowledge of the early universe contains some gaps. The cosmic microwave background reveals the intensity and polarization of primordial light as it was 380,000 years after the Big Bang, when the universe became transparent to light. We therefore cannot use electromagnetic radiation to directly study the universe before this time, although fluctuations in the temperature and polarization of the microwave background on very large scales do preserve much earlier events that took place during cosmic inflation. (Courtesy: NASA)
.
The first evidence for the primordial B-mode polarization of the cosmic microwave background (CMB) has been detected by astronomers working on the Background Imaging of Cosmic Extragalactic Polarization (BICEP2) telescope at the South Pole. The polarization signal is the first direct evidence for cosmic inflation and has been measured to a statistical certainty of 3σ. The primordial B-mode polarization is related to primordial gravitational waves that are thought to have abounded in the early universe.
Cosmologists believe that when the universe was very young – a mere 10–35 s after the Big Bang – it underwent a period of extremely rapid expansion, known as "inflation", when its volume increased by a factor of up to 1080 in a tiny fraction of a second. About 380,000 years after the Big Bang, the CMB – the thermal remnant of the Big Bang – came into being. Over the years, the CMB has been measured with great accuracy, but these observations brought some problems: the CMB showed that the entire observable universe seemed to be homogeneous, flat and isotropic, while the physics of the Big Bang suggests that it should be highly curved and heterogeneous.
Inflation was first proposed in 1980 by the physicists Alan Guth and Andrei Linde, and is currently the best way to resolve these conundrums. It suggests that the entire observable universe originated in a small causally connected region that expanded at an exponential rate so that the horizon became much larger and space could be flat. Inflation also serves the purpose of ironing out any anisotropies or curvature of space, putting the universe into a rather simple state that is only mildly pertubated by quantum fluctuations. Such fluctuations are thought to have occurred in a "microscopic inflationary region" that eventually magnified to cosmic size, becoming the seeds for the growth of structure in the universe – everything from stars to galaxies. There are also variations in temperature of about 100 µK in the CMB (which is normally at an even temperature of 3 K), which reveal density fluctuations in the early universe.
Scientists also believe that rather extreme gravitational conditions prevailed during the universe's infancy. Gargantuan primordial gravitational waves are thought to have propagated through the universe during the first moments of inflation and this would have produced a so-called cosmic gravitational-wave background (CGB). This gravitational-wave background would, in turn, have left its own imprint on the polarization of the CMB – a sort of "curl" component or rotation that is known as the primordial B-mode polarization.
.
The BICEP2 telescope is the white dish at the top right of the blue building. The dish on the left is the South Pole Telescope. (Courtesy: National Science Foundation)
.
This is why researchers the world over have been keen to detect the primordial B-mode polarization – it would be evidence of primordial gravitational waves and so inflation. And it is this B-mode polarization that the BICEP2 collaboration has detected for the first time. There is also another similar component known as non-primordial B-mode polarization, which is caused by gravitational lensing, and this was detected last year by the South Pole Telescope (SPT). There are also other polarization variations, known as E-mode or gradient variations, that describe how the magnitude of polarization changes over the CMB.
Scientists cannot distinguish between the polarization caused by gravitational waves, which has a tensor component, and that caused by density waves, which have a scalar component, simply by looking at the temperature variations of the CMB. Also, the primordial B-mode polarization is thought to be much weaker than the E-mode, making it even more difficult to detect. However, certain measurements on the polarization angles that can be detected at each point on the sky provide extra information and allow scientists to differentiate between the tensor and scalar components, providing a "tensor-to-scalar" ratio. This ratio has been measured by BICEP2 to be 0.20 with a statistical significant of about 3σ. The possibility that the ratio is zero is ruled out with a statistical certainty of 7σ.
A value of 0.20 is considered to be significantly larger than that expected from previous analyses of data from the Planck and WMAP telescopes. "This has been like looking for a needle in a haystack, but instead we found a crowbar," says BICEP2 co-leader Clem Pryke of the University of Minnesota. Craig Hogan, director of the Fermilab Center for Particle Astrophysics, told physicsworld.com that "If it's confirmed, it is truly profound – the first direct evidence not only for inflation, but of a quantum behaviour of space and time. The image of polarization is a relic imprint of roughly a single quanta of graviton action."
According to the BICEP2 collaboration, its results suggest that "the long search for tensor B-modes is apparently over, and a new era of B-mode cosmology has begun".
The BICEP2 results are available here.
In this video Andrew Jaffe of Imperial College London explains why cosmologists believe that the universe underwent a period of vast and raid growth when it was just fractions of a second old.
Quelle: Physics World
.
Update: 18.03.2014
.
Einstein's ripples: Your guide to gravitational waves
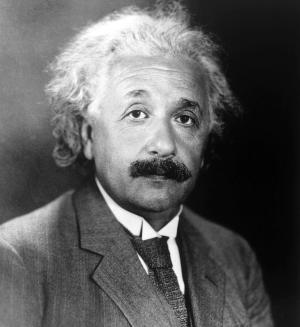
Hey Einstein, surf's up! Researchers from the BICEP2 telescope in Antarctica today announced the clearest detection yet of gravitational waves, ripples in space-time first predicted by the iconic physicist. The particular ripples the team detected were caused by inflation, the period immediately after the big bang, giving us a glimpse further back in our universe's history than ever before. That should help us piece together the exact sequence of events that led to our universe's birth, as well as a collection of other cosmic secrets. But what exactly is a ripple in space-time, anyway?
What are gravitational waves?
Long predicted but never directly seen, gravitational waves are ripples in the fabric of the universe.
Albert Einstein compared the universe's shape to a single fabric, hewn from space and time. According to his theory of general relativity, the force of gravity is the result of curvature in this space-time: gravitational waves are ripples in it. The ripples are produced by accelerating objects, just as an accelerating electric charge emits electromagnetic waves.
Colliding black holes and stars create modern-day gravitational waves. But cosmologists also believe the big bang itself produced primordial waves that still reverberate through space-time. It is these ripples, dating back to a fraction of a second after the birth of the universe, that the BICEP2 researchers have detected.
(Gravitational waves should not be confused with gravity waves, a term used in the study of fluids to refer to waves on a surface that propagate because of gravity.)
If the big bang's waves have been hanging around for so long, why haven't we found them until now?
Despite being produced by massive disturbances in space-time, even the largest gravitational waves are tiny. BICEP2 detected them by looking for patterns in the cosmic microwave background, the remnants of the first light in the universe. Gravitational waves squeeze space-time as they pass, leaving a small but meaningful fingerprint in this first light that the researchers could detect, but only after years of data analysis.
Has anyone tried to see them before?
BICEP2 has seen the first evidence for primordial gravitational waves – but the telltale signs of modern-day waves have been glimpsed before. In 1974, astronomers Russell Hulse and Joseph Taylor detected a binary pulsar, a pair of two dead stars emitting pulses of radio waves. Hulse and Taylor realised that the two pulsars were losing energy and slowly spiralling towards each other in a way that was exactly consistent with Einstein's equations of general relativity: the missing energy is thought to be emitted as gravitational waves. The finding earned the pair the Noble prize for physics in 1993.
If we've found modern gravitational waves, and primordial ones, is the hunt now over?
Not quite. Physicists still hope to surf a passing gravitational wave, which would amount to the first direct detection of these entities, in contrast to the indirect effects seen by BICEP2 and Hulse and Taylor.
So how do you surf a gravitational wave?
Like light waves, gravitational waves come in many different frequencies depending on how they are produced (see diagram, below).
.
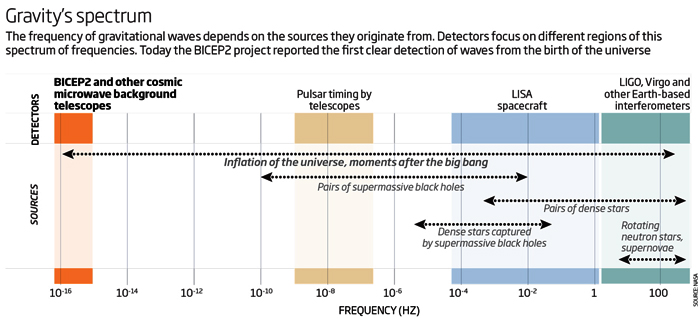
Experiments like LIGO, which is spread over two sites in Washington state and Louisiana in the US, and VIRGO, in Cascina, Italy, look for high-frequency waves, caused by modern-day black hole mergers or exploding stars. These should cause slight disturbances on Earth that can be measured – although none of the detectors has yet reported such an event.
Detectors in space, meanwhile, can detect lower frequency waves – next year the European Space Agency plans to launch LISA Pathfinder, a test mission that will lead to a trio of spacecraft in 2034 intended to hunt for gravitational waves emitted by black holes.
Quelle: NewScientist