.
4.03.2014
The sky as seen in gamma rays from the Fermi telescope. The red line through the center is the disk of our galaxy. Image: NASA/DOE/Fermi LAT Collaboration
.
The more that scientists stare at it, the more a strange signal from the center of the Milky Way galaxy appears to be the result of dark matter annihilation. If confirmed, it would be the first direct evidence for dark matter ever seen.
Dark matter is a mysterious, invisible substance making up roughly 85 percent of all matter in the universe. It floats throughout our galaxy, but is more concentrated at its center. There, a dark matter particle can meet another dark matter particle flying through space. If they crash into one another, they will annihilate each other (dark matter is its own antiparticle) and give off gamma rays.
To search for a dark matter signal, astronomers use NASA’s Fermi Gamma-Ray Telescope to map the gamma radiation throughout the galaxy. Then, they try to account for all known sources of light within this map. They plot the location of gas and dust that could be emitting radiation and subtract that signal from their gamma-ray map. Then they determine where all the stars are and subtract out that light, and so on for every object that might be emitting radiation. Once all those sources are gone, there remains a tiny excess of gamma radiation in the data that no known process can account for.
“The more we scrutinize it, the more it looks like dark matter,” said astrophysicist Dan Hooper of Fermi National Accelerator Laboratory, co-author of a paper that appeared Feb. 26 on arXiv, a website that hosts scientific papers that have yet to go through peer-review.
Since 2009, Hooper has been claiming that this bright signal is evidence of dark matter. According to his team’s latest data, the gamma radiation could be produced by dark matter particles with a mass of 30 to 40 gigaelectronvolts (GeV) crashing into one another. A proton is roughly 1 GeV for comparison.
But the galactic center is a tricky place. There are many other gamma ray sources that could be mimicking a dark matter signal as well as yet undiscovered phenomena that might account for the radiation. For the most part, few other researchers have been convinced of Hooper’s data. One oft-used counterargument is that the excess gamma ray signal could come from millisecond pulsars — dead star cores that spin extremely fast and beam out a huge amount of energy. Astronomers don’t yet have a good understanding of how these objects work.
“If you need to explain something weird in the galactic center, you wave your hands and say, ‘Millisecond pulsars,’” said astronomer Doug Finkbeiner of Harvard, another co-author of the new work.
Finkbeiner has long been a skeptic that the excess Fermi telescope signal represents dark matter annihilation. He knows that the galactic center is a strange place full of unexpected phenomena, having discovered in 2010 two gigantic structures spanning 50,000 light-years emanating from the Milky Way, which had gone unnoticed until then. But a more careful look at Hooper’s data has started to convince Finkbeiner that there might be something there.
When a galaxy forms, gravitational attraction brings together a huge mass that begins spinning. As they spin, large galaxies cool down and flatten out like a pizza, forming the familiar spiral shape seen in many telescope images. Dark matter, which actually makes up the bulk of a galaxy’s mass, can’t flatten out because it doesn’t interact with the electromagnetic force, which would allow it to radiate away thermal energy. It stays in a spherical halo circling the galaxy. So any dark matter signal should come not just from within the galactic plane, but also from above and below it, where stars are few and far between but dark matter is abundant.
The problem is that the galactic center is extremely bright. Its billions of stars give off an incredible amount of light that shines far above and below the plane of the Milky Way. Showing that the gamma ray signal comes from dark matter and nothing else requires extremely precise mapping. But the Fermi telescope’s data also happens to be a little blurry at the energy ranges where the dark matter signal shows up. Working with physicist Tracy Slatyer of MIT, Finkbeiner combed through the Fermi data and found a way to throw out the blurriest parts. This left a very sharp map showing exactly that the excess gamma ray signal was coming from areas where few stars should exist.
“The answers just got a lot better,” said Finkbeiner. “It looked more like dark matter and less like pulsars.”
The newly sharpened data is making other researchers take notice. “We may in the future say this was when dark matter was discovered,” said theoretical physicist Neal Weiner of New York University.
A particle mass of 30 to 40 GeV would make this form of dark matter quite interesting, he added, because it is something that could have shown up at the Large Hadron Collider. The fact that it didn’t might suggest that dark matter is more complicated than our simplest models predict.
But others remain more skeptical. “If the question is, ‘Have we really discovered dark matter?’ I would really caution to set the burden of proof as high as we can,” said physicist Stefano Profumo of the University of California, Santa Cruz.
There are many things that our Earth-bound perspective might not be taking into account, such as differences in the density or energies of cosmic rays in the galactic center, he added.
Even though he is a co-author of the new work, Finkbeiner also remains cautious. Of all the options he is aware of, he thinks dark matter annihilation remains the best explanation for the Fermi telescope’s excess signal. But the universe is vast and there likely remain many unknown objects for astronomers to find.
To definitively answer this question, scientists will likely have to study dwarf galaxies, which are up to 99 percent dark matter and contain few other odd phenomena that could mimic a dark matter signal. The Fermi telescope will have to stare at these objects for a few more years before it has enough data to confirm or deny the latest results.
Quelle:WIRED
.
Update: 5.03.2014
.
Case for Dark Matter Signal Strengthens
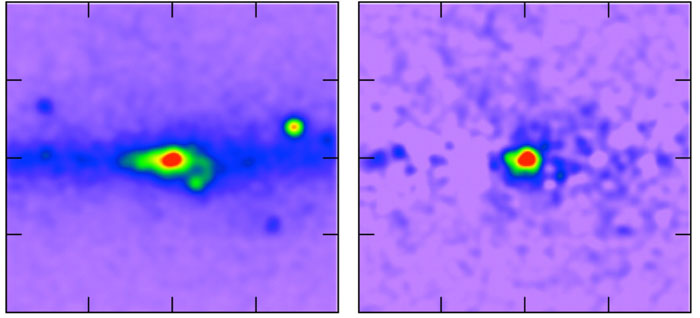
Maps of gamma rays from the center of the Milky Way galaxy, before (left) and after signals from known sources were removed, reveal an excess that is consistent with the distribution of dark matter.
.
Not long after the Fermi Gamma-ray Space Telescope took to the sky in 2008, astrophysicists noticed that it was picking up a steady rain of gamma rays pouring outward from the center of the Milky Way galaxy. This high-energy radiation was consistent with the detritus of annihilating dark matter, the unidentified particles that constitute 84 percent of the matter in the universe and that fizzle upon contact with each other, spewing other particles as they go. If the gamma rays did in fact come from dark matter, they would reveal its identity, resolving one of the biggest mysteries in physics. But some argued that the gamma rays could have originated from another source.
Now a new analysis of the signal claims to rule out all other plausible explanations and makes the case that the gamma rays trace back to a type of particle that has long been considered the leading dark matter candidate — a weakly interacting massive particle, or WIMP. Meanwhile, a more tentative X-ray signal reported in two other new studies suggests the existence of yet another kind of dark matter particle called a sterile neutrino.
In the new gamma-ray analysis, which appeared Feb. 27 on the scientific preprint site arXiv.org, Dan Hooper and his collaborators used more than five years’ worth of the cleanest Fermi data to generate a high-resolution map of the gamma-ray excess extending from the center of the galaxy outward at least 10 angular degrees, or 5,000 light-years, in all directions.
“The results are extremely interesting,” said Kevork Abazajian, an associate professor of physics and astronomy at the University of California, Irvine. “The most remarkable part of the analysis is that the signal follows the shape of the dark matter profile out to 10 degrees,” he said, explaining that it would be “very difficult to impossible” for other sources to mimic this predicted dark matter distribution over such a broad range.
The findings do not constitute a discovery of dark matter, the scientists said, but they prepare the way for an upcoming test described by many researchers as a “smoking gun”: If the gamma-ray excess comes from annihilating WIMPs, and not conventional astrophysical objects, then the signal will also be seen emanating from dwarf galaxies that orbit the Milky Way — diffuse objects that are rich in dark matter but not in other high-energy photon sources such as pulsars, rotating neutron stars that have been floated as alternative explanations for the excess.
“These gamma rays match the predictions of a pretty prototypical WIMP, the kind of thing we were all writing down 10 or 15 years ago,” said Hooper, a theoretical astrophysicist at the Fermi National Accelerator Laboratory and the University of Chicago, and the person who co-discovered the gamma-ray excess with then graduate student Lisa Goodenough in 2009. “That’s where my money is.”
“It’s definitely exciting,” said Neal Weiner, a dark matter specialist at New York University. “I think we’d like to see it somewhere else, like a dwarf galaxy, before getting really excited.”
Preliminary results from the Fermi Collaboration — scientists who process, analyze and release the telescope data — offer hints that there may indeed be a surplus of gamma rays coming from the dwarf galaxies. Although there is currently too little data to determine whether an excess exists, “we are starting to get closer to the range,” said Jennifer Siegal-Gaskins, a physicist at the California Institute of Technology and a member of the Fermi Collaboration. “I would say the next couple of years of data could really be important for testing this excess.”
“If that small excess from the dwarf galaxies turns to a big one, that would convince the whole community,” Hooper said. “That would be game over.”
While most experts agree, some question whether indirect glimpses of dark matter can ever truly constitute a discovery.
Dark matter consists of elementary particles that do not emit or absorb light, because they do not experience the electromagnetic force. These particles are also unaffected by the strong nuclear force, which ensnares many of the known particles into atoms. Cosmologists infer the existence of dark matter, and can model its distribution throughout the cosmos, because it does participate in the force of gravity and therefore plays a leading role in shaping galaxies. If dark matter particles also experience the fourth and final force of nature, called the weak nuclear force, then they are of a type known as a WIMP.
In many theories, pairs of WIMPs can annihilate each other on contact, emitting other particles as they go. If the glimmer of gamma rays from the inner galaxy is the afterglow from such annihilations, then their detected energy levels indicate that they most likely originate from WIMPs with a mass of 35 giga-electron-volts (GeV) annihilating into quarks, or 10-GeV WIMPs annihilating into tau particles.
The 35-GeV WIMP model “fits the data best,” said Tracy Slatyer, an assistant professor of physics at the Massachusetts Institute of Technology and a co-author of the new paper. The fit has greatly improved, she said, since the group’s last analysis of the gamma-ray excess. If the signal wasn’t from dark matter, “it’s not at all clear that going to a better data sample would make the results look better,” she said, “but when I saw the new results, I was amazed.”
WIMPs have not yet shown up in direct detection experiments, which look for spurts of energy coming from their weak interactions with atomic nuclei, usually in detectors placed in mine shafts deep underground to lower the background noise. But this does not mean 35-GeV WIMPs don’t exist, scientists said, because no one knows how frequently they interact. The authors of the new study “could be perfectly right, and we just need detectors two orders of magnitude more sensitive to see the particles,” said Juan Collar, an associate professor of physics at the University of Chicago who helps develop direct detection experiments.
Most of the researchers interviewed for this article said the presence of a gamma-ray excess from the dwarf galaxies would be sufficient proof of WIMPs, but a few said that it might take a direct detection to convince them. “The problem is the universe is a messy place,” said Kathryn Zurek, an associate professor of physics at the University of Michigan. Try as they might to rule out “astrophysics” — shorthand among dark matter researchers for all the conventional stuff in the sky, from pulsars to supernovae to the sun — it is always possible that they have missed something.
The study authors, however, are confident that dark matter is the only plausible source of the gamma rays. “We threw everything including the kitchen sink at the problem,” Hooper said. “My views are on the record.”
Meanwhile, just as Hooper’s group was putting the finishing touches on the new manuscript, two other teams of scientists independently reported the discovery of a different anomaly in the sky: a dash of X-rays emanating from distant galaxies that is consistent with the decay of 7-kilo-electron-volt (keV) sterile neutrinos — heavier and less active cousins of the familiar neutrinos that are also dark matter candidates.
Esra Bulbul, a postdoctoral fellow at the Harvard-Smithsonian Center for Astrophysics, and her colleagues spotted the X-rays in data from the Chandra and XMM-Newton space telescopes and published their results Feb. 10. A week later, a group led by Alexey Boyarsky of the University of Leiden in the Netherlands reported the same X-ray excess in telescope observations of the Andromeda galaxy.
“I think we have a very big fish here,” Bulbul said.
Bulbul and colleagues report a statistical significance of between 4 and 5 sigma, meaning the X-ray signal is strong enough that the odds that it is a random fluke are only one in 100,000. However, putative dark matter signals often hover at the 4-sigma brink of statistical significance only to fade into the background when more data is collected. Seasoned veterans of this boom-and-bust cycle are skeptical about the new anomaly, but some have expressed cautious optimism.
“It’s definitely intriguing,” said John Beacom, a theoretical astrophysicist at Ohio State University. “They certainly have tried very hard to eliminate or examine the possibility of an atomic transition being the cause. They’ve also gone to great lengths to eliminate instrumental effects.”
The X-ray bump appeared in all subsets of the data, no matter how Bulbul and colleagues sliced it — a sign that the bump did not come from a bias somewhere in the telescope instrumentation. It was this same omnipresence that convinced particle physicists at the Large Hadron Collider that they had cornered the Higgs boson in 2012 before their signal reached the 5-sigma strength formally needed for a discovery. Further support for the significance of the X-ray excess comes from the Dutch group’s discovery of the same bump at 4.4-sigma strength in a different data set.
If the X-rays come from sterile neutrinos, the existence of these particles would very likely solve a long-standing puzzle about galaxy formation known as the “too big to fail” problem, which asks why objects called dark matter subhalos don’t collapse and form dwarf galaxies. “That’s one of the reasons I’m actually more excited about this result than I would be otherwise,” Abazajian said. The particles also play a role in the seesaw mechanism, the most widely supported explanation for the minuscule mass of regular neutrinos. Decays of sterile neutrinos shortly after the Big Bang could even explain the mysterious dominance of matter over antimatter in the universe today. “Sterile neutrinos get invoked for twenty different reasons,” Beacom said.
Like the gamma-ray signal, the X-ray excess will face a clear-cut test in the near future. The Astro-H telescope, set for launch in 2015, will be sensitive enough to detect the smear of the signal. If the width of the bump is consistent with the expected speed of decaying dark matter particles, “that would be a detection,” Abazajian said.
Both signals are tough to dismiss, raising a strange prospect. “It’s possible they are both dark matter,” Abazajian said. “It would be crazy, but it’s certainly possible.”
The sterile neutrinos associated with the X-rays could account for anywhere from 1 to 100 percent of dark matter, depending on how often they decay. And the WIMPs tied to the gamma rays are almost as flexible. The two could coexist. As Collar put it, “If the matter we know about is so rich in families of particles, what tells you this dark sector we know nothing about is not as rich or richer?”
A theoretical model called “exciting dark matter,” proposed in 2007 by Weiner and Douglas Finkbeiner of Harvard, a co-author of the new gamma-ray paper, even predicts the existence of both a keV-scale dark matter particle and a GeV-scale particle working in tandem. “So, at the moment, I’m quite excited!” Weiner said in an email.
But at least for the next couple of years, another nagging possibility remains.
“It’s like the Monty Python sketch — nobody ever expects the Spanish Inquisition,” Beacom said. “Sometimes in this field nobody expects astrophysics, but it’s almost always astrophysics. All of these groups have tried to be very careful, but it is difficult, and nature may surprise us with astrophysics yet again.”
Quelle: QUANTA-MAGAZINE
5284 Views