Scientists at MIT, the University of Birmingham, and elsewhere say that astronomers’ best chance of finding liquid water, and even life on other planets, is to look for the absence, rather than the presence, of a chemical feature in their atmospheres.
The researchers propose that if a terrestrial planet has substantially less carbon dioxide in its atmosphere compared to other planets in the same system, it could be a sign of liquid water — and possibly life — on that planet’s surface.
What’s more, this new signature is within the sights of NASA’s James Webb Space Telescope (JWST). While scientists have proposed other signs of habitability, those features are challenging if not impossible to measure with current technologies. The team says this new signature, of relatively depleted carbon dioxide, is the only sign of habitability that is detectable now.
“The Holy Grail in exoplanet science is to look for habitable worlds, and the presence of life, but all the features that have been talked about so far have been beyond the reach of the newest observatories,” says Julien de Wit, assistant professor of planetary sciences at MIT. “Now we have a way to find out if there’s liquid water on another planet. And it’s something we can get to in the next few years.”
The team’s findings appear today in Nature Astronomy. De Wit co-led the study with Amaury Triaud of the University of Birmingham in the UK. Their MIT co-authors include Benjamin Rackham, Prajwal Niraula, Ana Glidden Oliver Jagoutz, Matej Peč, Janusz Petkowski, and Sara Seager, along with Frieder Klein at the Woods Hole Oceanographic Institution (WHOI), Martin Turbet of Ècole Polytechnique in France, and Franck Selsis of the Laboratoire d’astrophysique de Bordeaux.
Beyond a glimmer
Astronomers have so far detected more than 5,200 worlds beyond our solar system. With current telescopes, astronomers can directly measure a planet’s distance to its star and the time it takes it to complete an orbit. Those measurements can help scientists infer whether a planet is within a habitable zone. But there’s been no way to directly confirm whether a planet is indeed habitable, meaning that liquid water exists on its surface.
Across our own solar system, scientists can detect the presence of liquid oceans by observing “glints” — flashes of sunlight that reflect off liquid surfaces. These glints, or specular reflections, have been observed, for instance, on Saturn’s largest moon, Titan, which helped to confirm the moon’s large lakes.
Detecting a similar glimmer in far-off planets, however, is out of reach with current technologies. But de Wit and his colleagues realized there’s another habitable feature close to home that could be detectable in distant worlds.
“An idea came to us, by looking at what’s going on with the terrestrial planets in our own system,” Triaud says.
Venus, Earth, and Mars share similarities, in that all three are rocky and inhabit a relatively temperate region with respect to the sun. Earth is the only planet among the trio that currently hosts liquid water. And the team noted another obvious distinction: Earth has significantly less carbon dioxide in its atmosphere.
“We assume that these planets were created in a similar fashion, and if we see one planet with much less carbon now, it must have gone somewhere,” Triaud says. “The only process that could remove that much carbon from an atmosphere is a strong water cycle involving oceans of liquid water.”
Indeed, the Earth’s oceans have played a major and sustained role in absorbing carbon dioxide. Over hundreds of millions of years, the oceans have taken up a huge amount of carbon dioxide, nearly equal to the amount that persists in Venus’ atmosphere today. This planetary-scale effect has left Earth’s atmosphere significantly depleted of carbon dioxide compared to its planetary neighbors.
“On Earth, much of the atmospheric carbon dioxide has been sequestered in seawater and solid rock over geological timescales, which has helped to regulate climate and habitability for billions of years,” says study co-author Frieder Klein.
The team reasoned that if a similar depletion of carbon dioxide were detected in a far-off planet, relative to its neighbors, this would be a reliable signal of liquid oceans and life on its surface.
“After reviewing extensively the literature of many fields from biology, to chemistry, and even carbon sequestration in the context of climate change, we believe that indeed if we detect carbon depletion, it has a good chance of being a strong sign of liquid water and/or life,” de Wit says.
A roadmap to life
In their study, the team lays out a strategy for detecting habitable planets by searching for a signature of depleted carbon dioxide. Such a search would work best for “peas-in-a-pod” systems, in which multiple terrestrial planets, all about the same size, orbit relatively close to each other, similar to our own solar system. The first step the team proposes is to confirm that the planets have atmospheres, by simply looking for the presence of carbon dioxide, which is expected to dominate most planetary atmospheres.
“Carbon dioxide is a very strong absorber in the infrared, and can be easily detected in the atmospheres of exoplanets,” de Wit explains. “A signal of carbon dioxide can then reveal the presence of exoplanet atmospheres.”
Once astronomers determine that multiple planets in a system host atmospheres, they can move on to measure their carbon dioxide content, to see whether one planet has significantly less than the others. If so, the planet is likely habitable, meaning that it hosts significant bodies of liquid water on its surface.
But habitable conditions doesn’t necessarily mean that a planet is inhabited. To see whether life might actually exist, the team proposes that astronomers look for another feature in a planet’s atmosphere: ozone.
On Earth, the researchers note that plants and some microbes contribute to drawing carbon dioxide, although not nearly as much as the oceans. Nevertheless, as part of this process, the lifeforms emit oxygen, which reacts with the sun’s photons to transform into ozone — a molecule that is far easier to detect than oxygen itself.
The researchers say that if a planet’s atmosphere shows signs of both ozone and depleted carbon dioxide, it likely is a habitable, and inhabited world.
“If we see ozone, chances are pretty high that it’s connected to carbon dioxide being consumed by life,” Triaud says. “And if it’s life, it’s glorious life. It would not be just a few bacteria. It would be a planetary-scale biomass that’s able to process a huge amount of carbon, and interact with it.”
The team estimates that NASA’s James Webb Space Telescope would be able to measure carbon dioxide, and possibly ozone, in nearby, multiplanet systems such as TRAPPIST-1 — a seven-planet system that orbits a bright star, just 40 light years from Earth.
“TRAPPIST-1 is one of only a handful of systems where we could do terrestrial atmospheric studies with JWST,” de Wit says. “Now we have a roadmap for finding habitable planets. If we all work together, paradigm-shifting discoveries could be done within the next few years.”
Quelle: MIT Massachusetts Institute of Technology
----
Update: 11.01.2024
.
NASA’s Webb Finds Signs of Possible Aurorae on Isolated Brown Dwarf
Infrared emission from methane suggests atmospheric heating by auroral processes.
Astronomers using NASA’s James Webb Space Telescope have found a brown dwarf (an object more massive than Jupiter but smaller than a star) with infrared emission from methane, likely due to energy in its upper atmosphere. This is an unexpected discovery because the brown dwarf, W1935, is cold and lacks a host star; therefore, there is no obvious source for the upper atmosphere energy. The team speculates that the methane emission may be due to processes generating aurorae.
These findings are being presented at the 243rd meeting of the American Astronomical Society in New Orleans.
To help explain the mystery of the infrared emission from methane, the team turned to our solar system. Methane in emission is a common feature in gas giants like Jupiter and Saturn. The upper-atmosphere heating that powers this emission is linked to aurorae.
Image: Artist Concept Brown Dwarf W1935
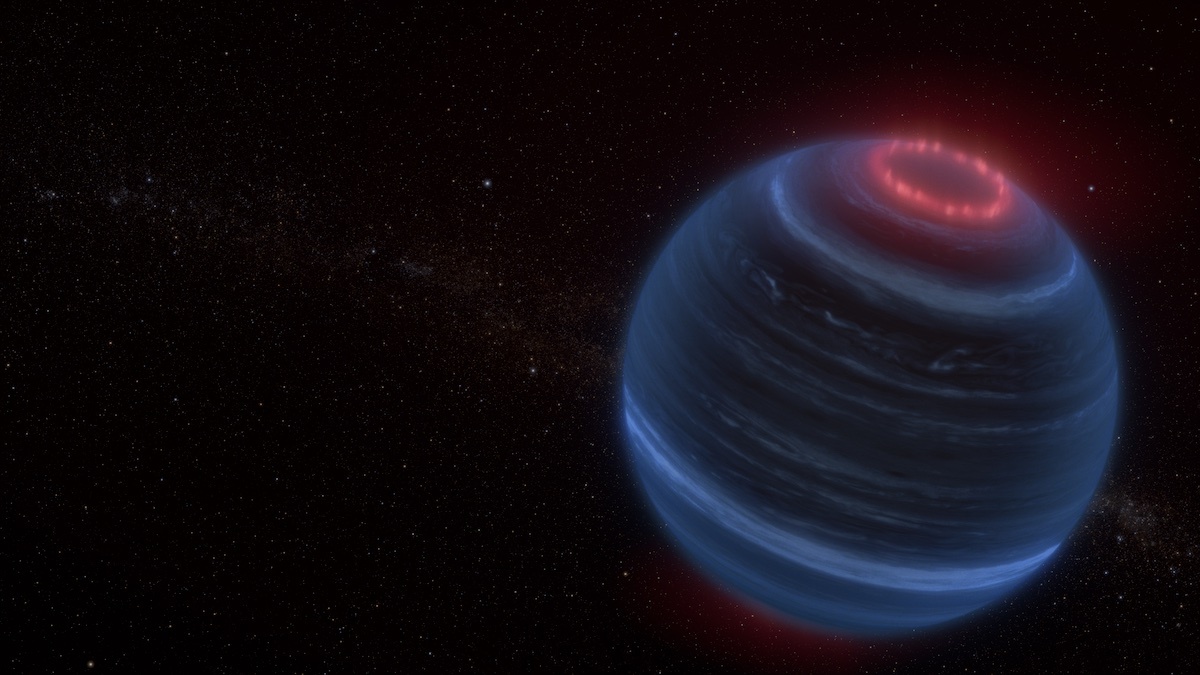
This artist concept portrays the brown dwarf W1935, which is located 47 light-years from Earth. Astronomers using NASA’s James Webb Space Telescope found infrared emission from methane coming from W1935. This is an unexpected discovery because the brown dwarf is cold and lacks a host star; therefore, there is no obvious source of energy to heat its upper atmosphere and make the methane glow. The team speculates that the methane emission may be due to processes generating aurorae, shown here in red.
NASA, ESA, CSA, and L. Hustak (STScI)
On Earth, aurorae are created when energetic particles blown into space from the Sun are captured by Earth’s magnetic field. They cascade down into our atmosphere along magnetic field lines near Earth’s poles, colliding with gas molecules and creating eerie, dancing curtains of light. Jupiter and Saturn have similar auroral processes that involve interacting with the solar wind, but they also get auroral contributions from nearby active moons like Io (for Jupiter) and Enceladus (for Saturn).
For isolated brown dwarfs like W1935, the absence of a stellar wind to contribute to the auroral process and explain the extra energy in the upper atmosphere required for the methane emission is a mystery. The team surmises that either unaccounted internal processes like the atmospheric phenomena of Jupiter and Saturn, or external interactions with either interstellar plasma or a nearby active moon, may help account for the emission.
A Detective Story
The aurorae’s discovery played out like a detective story. A team led by Jackie Faherty, an astronomer at the American Museum of Natural History in New York, was awarded time with the Webb telescope to investigate 12 cold brown dwarfs. Among those were W1935 – an object that was discovered by citizen scientist Dan Caselden, who worked with the Backyard Worlds zooniverse project – and W2220, an object that was discovered using NASA’s Wide Field Infrared Survey Explorer. Webb revealed in exquisite detail that W1935 and W2220 appeared to be near clones of each other in composition. They also shared similar brightness, temperatures, and spectral features of water, ammonia, carbon monoxide, and carbon dioxide. The striking exception was that W1935 showed emission from methane, as opposed to the anticipated absorption feature that was observed toward W2220. This was seen at a distinct infrared wavelength to which Webb is uniquely sensitive.
“We expected to see methane because methane is all over these brown dwarfs. But instead of absorbing light, we saw just the opposite: The methane was glowing. My first thought was, what the heck? Why is methane emission coming out of this object?” said Faherty.
The team used computer models to infer what might be behind the emission. The modeling work showed that W2220 had an expected distribution of energy throughout the atmosphere, getting cooler with increasing altitude. W1935, on the other hand, had a surprising result. The best model favored a temperature inversion, where the atmosphere got warmer with increasing altitude. “This temperature inversion is really puzzling,” said Ben Burningham, a co-author from the University of Hertfordshire in England and lead modeler on the work. “We have seen this kind of phenomenon in planets with a nearby star that can heat the stratosphere, but seeing it in an object with no obvious external heat source is wild.”
Image: Spectra W1935 vs W2220
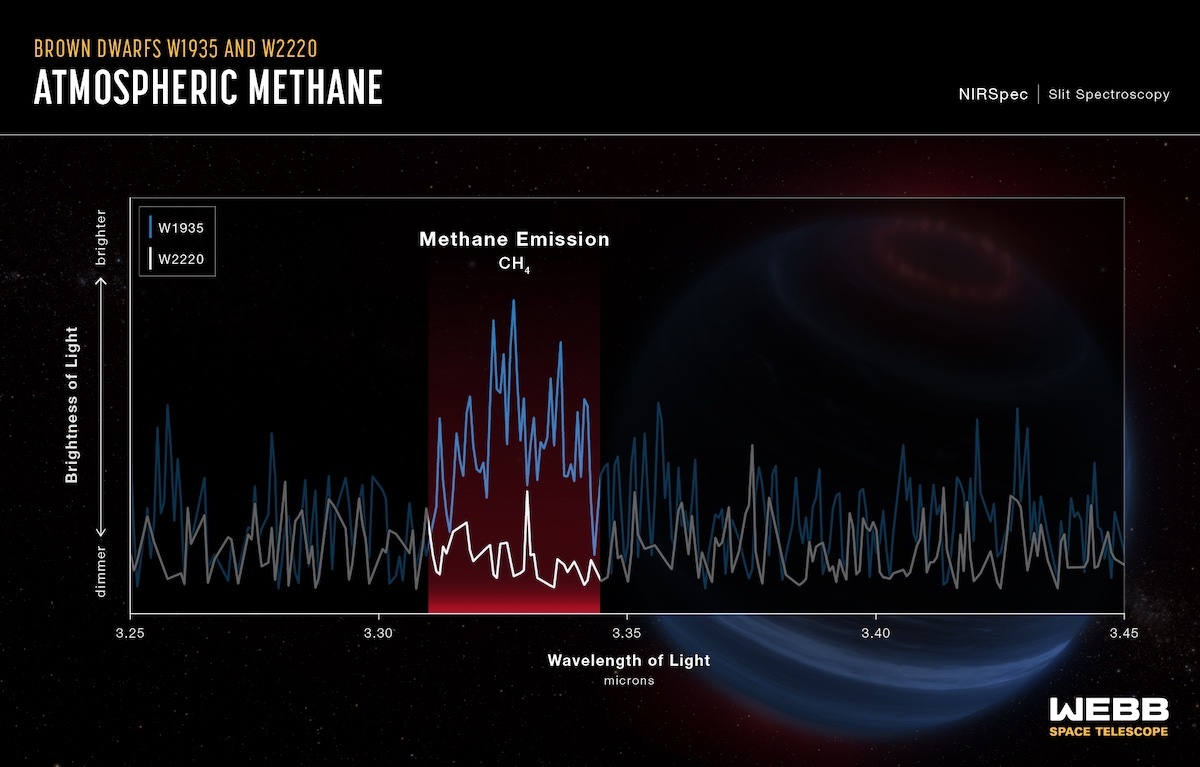
Astronomers used NASA’s James Webb Space Telescope to study 12 cold brown dwarfs. Two of them – W1935 and W2220 – appeared to be near twins of each other in composition, brightness, and temperature. However, W1935 showed emission from methane, as opposed to the anticipated absorption feature that was observed toward W2220. The team speculates that the methane emission may be due to processes generating aurorae.
NASA, ESA, CSA, and L. Hustak (STScI)
Clues from our Solar System
For clues, the team looked in our own backyard, to the planets of our solar system. The gas giant planets can serve as proxies for what is seen going on more than 40 light-years away in the atmosphere of W1935.
The team realized that temperature inversions are prominent in planets like Jupiter and Saturn. There is still ongoing work to understand the causes of their stratospheric heating, but leading theories for the solar system involve external heating by aurorae and internal energy transport from deeper in the atmosphere (with the former a leading explanation).
Brown Dwarf Aurora Candidates in Context
This is not the first time an aurora has been used to explain a brown dwarf observation. Astronomers have detected radio emission coming from several warmer brown dwarfs and invoked aurorae as the most likely explanation. Searches were conducted with ground-based telescopes like the Keck Observatory for infrared signatures from these radio-emitting brown dwarfs to further characterize the phenomenon, but were inconclusive.
W1935 is the first auroral candidate outside the solar system with the signature of methane emission. It’s also the coldest auroral candidate outside our solar system, with an effective temperature of about 400 degrees Fahrenheit (200 degrees Celsius), about 600 degrees Fahrenheit warmer than Jupiter.
In our solar system the solar wind is a primary contributor to auroral processes, with active moons like Io and Enceladus playing a role for planets like Jupiter and Saturn, respectively. W1935 lacks a companion star entirely, so a stellar wind cannot contribute to the phenomenon. It is yet to be seen whether an active moon might play a role in the methane emission on W1935.
“With W1935, we now have a spectacular extension of a solar system phenomenon without any stellar irradiation to help in the explanation.” Faherty noted. “With Webb, we can really ‘open the hood’ on the chemistry and unpack how similar or different the auroral process may be beyond our solar system,” she added.
The James Webb Space Telescope is the world’s premier space science observatory. Webb is solving mysteries in our solar system, looking beyond to distant worlds around other stars, and probing the mysterious structures and origins of our universe and our place in it. Webb is an international program led by NASA with its partners, ESA (European Space Agency) and the Canadian Space Agency.
Quelle: NASA
----
Update: 25.01.2024
.
Nearby star factory shines in stunning James Webb Space Telescope photo
The powerful space telescope captured a starburst complex in the Large Magellanic Cloud.
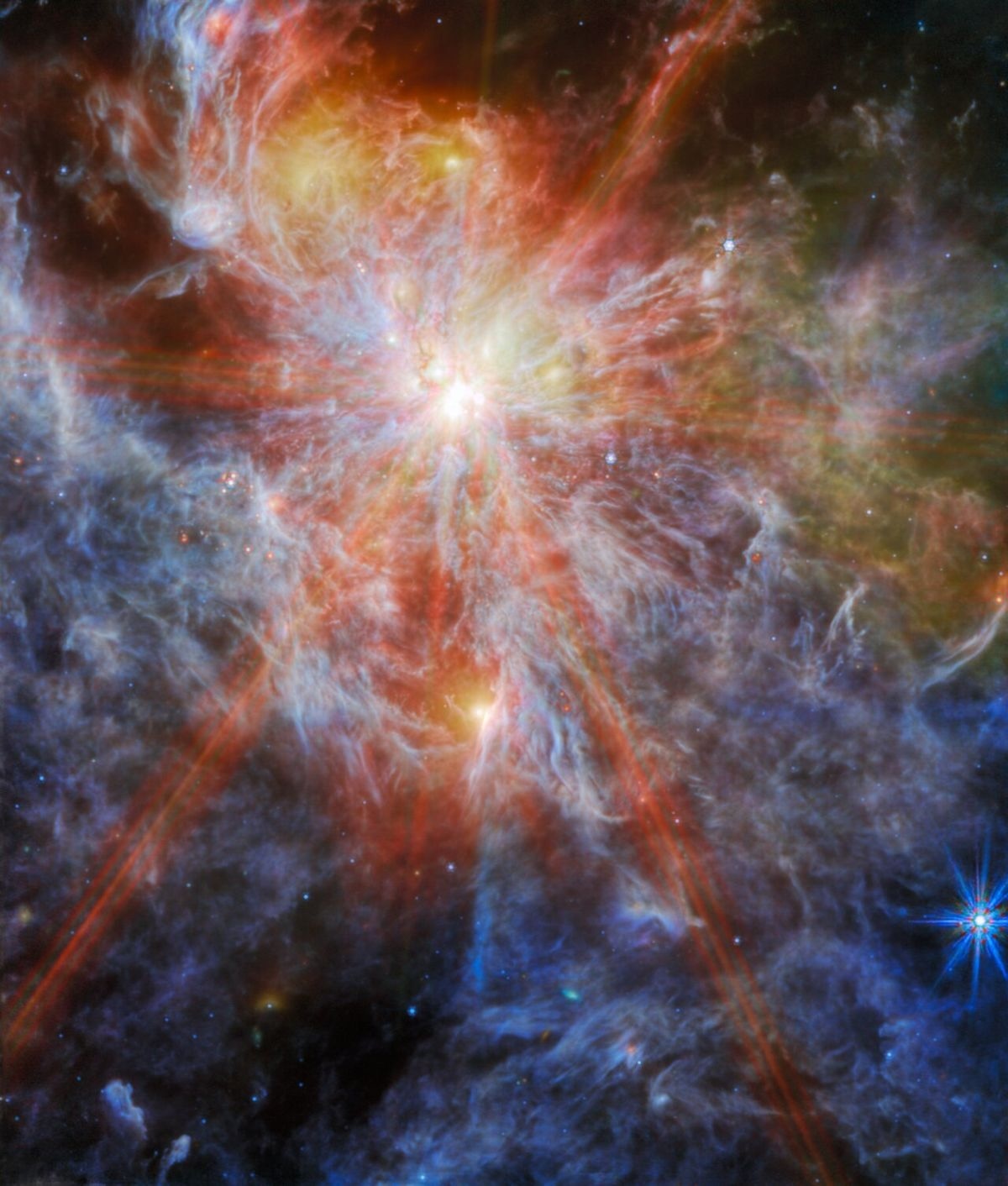
James Webb Space Telescope image of the stellar nursery N79 in the Large Magellanic Cloud, a satellite galaxy of the Milky Way. (Image credit: ESA/Webb, NASA & CSA, O. Nayak, M. Meixner)
A stunning new image from the James Webb Space Telescope (JWST) shows a vast star factory located in a neighboring galaxy in vibrant colors and incredible detail.
The orange, yellow and blue image from the powerful space telescope features the interstellar atomic hydrogen of the 1,630-light-year-wide nebula N79, located in the Large Magellanic Cloud, a satellite galaxy of the Milky Way. This region is actively forming stars and remains virtually unexplored by astronomers.
N79 is considered to be a younger sibling of another recent JWST target in the Large Magellanic Cloud, the Tarantula Nebula, which lies about 161,000 light-years from Earth.
Despite their similarities, scientists think that over the last 500,000 years, N79 has been forming stars at twice the rate of the Tarantula Nebula, officially known as 30 Doradus.
Studying regions of intense star birth like N79 with the JWST allows scientists to learn about the composition of star-birthing clouds of gas and dust in the early universe, when star formation was at its most intense.
The new JWST image focuses on three giant complexes of cold atomic gas called molecular clouds, which comprise what astronomers call N79 South, or S1.
One of the most striking aspects of the image is the "starburst" pattern that surrounds the bright heart of N79. This effect is created by diffraction spikes caused by the 18 pieces of JWST's primary mirror as they collect light. These mirrors are arranged in a hexagonal pattern like a honeycomb, meaning there are six main diffraction spikes.
These diffraction spikes arise when JWST studies particularly bright and compact objects that have light emerging from a concentrated location. So, when the $10 billion telescope looks at galaxies, even ones that may appear very small, the light comes from more diffuse and spread-out sources, meaning the diffraction pattern is absent.
JWST captured the new N79 image using its Mid-InfraRed Instrument (MIRI). Visible light is readily absorbed by such dense clouds of dust, but long-wave infrared light goes through more easily. So the infrared view of MIRI allows astronomers to peer deep into this star-forming region.
As a result, JWST is also able to see young stellar bodies that are still cocooned in their natal womb of gas and dust. These so-called "protostars" haven't yet gathered enough material from this envelope to become massive enough to fuse hydrogen to helium in their cores, the process that defines what a star is.
An infant star that has just started this process can be seen as the brightest point in the midst of billowing orange clouds of gas and dust in the JWST N97 image.
The JWST observations of N79 are part of the powerful telescope's mission, which includes (in part) examining the evolution of disks and envelopes of material surrounding stars at different stages of their evolution.
It is hoped that, as part of this mission, JWST will help astronomers get their first look at planet-forming disks of material surrounfing young stars that resemble the sun, thus providing them with a picture of how our solar system formed around 4.6 billion years ago.
Quelle: SC
----
Update: 26.01.2024
.
Modern age JWST image development techniques reveal faint features in galaxy NGC 5728
Mason Leist is working remotely — 127 million light-years from Earth — on images of a supermassive black hole in his office at the UTSA Department of Physics and Astronomy.
The UTSA graduate research assistant led a study, published in The Astronomical Journal, on the best method to improve images obtained by the James Webb Science Telescope (JWST) using a mathematical approach called deconvolution. He was tasked by the Galactic Activity, Torus, and Outflow Survey (GATOS), an international team of scientists, to enhance JWST observations of the galaxy NGC 5728.
The GATOS team, co-led by UTSA professor and Leist’s doctoral advisor Chris Packham, was awarded time on the JWST for its research on black holes.
“It’s incredibly humbling,” Leist said. “Not just working with JWST data, which is a great opportunity and a crazy amount of science, but working with our collaborators. It’s a very incredible experience to collaborate with other members of the GATOS on this. I like to tell people that this work represents the efforts of 35 individuals from institutes in 14 countries.”
“It’s a very incredible experience to collaborate with other members of the GATOS on this.”
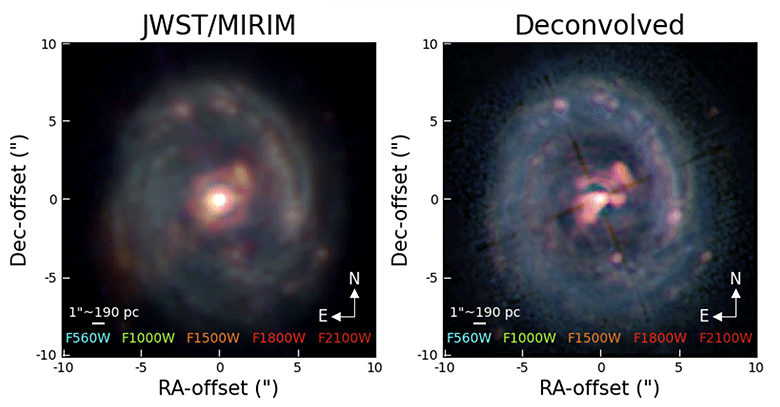
Images obtained by the James Webb Science Telescope that were improved using a mathematical approach called deconvolution.
Leist deconvolved simulated and observed images of an active galactic nucleus (AGN), a region at the center of the galaxy NGC 5728. The central engine of an active galactic nucleus, comprised of a hot and turbulent accretion disk orbiting a central supermassive black hole enshrouded by a thick torus of gas and dust, plays a key role in feedback between the AGN, host galaxy and intergalactic medium.
He tested five deconvolution algorithms over two years on simulated observations of an AGN. Of the five methods tested, the Kraken algorithm improved the simulated AGN model image quality the most and was therefore applied to JWST observations of NGC 5728. Kraken is a high-performance multi-frame deconvolution algorithm developed by a team of researchers led by Douglas Hope and Stuart Jefferies at Georgia State University.
JWST observed NGC 5728 at five distinct wavelengths. In these observations, a faint extended feature was seen in only one wavelength. As Leist deconvolved the data, the faint extended emission feature was revealed in all wavelengths, demonstrating the effectiveness of Kraken deconvolution to improve JWST image quality and enhance faint extended emission features.
“We believe the extension could be part of an outflow from a supermassive black hole that could be interacting with the host galaxy. There’s a lot more science that needs to be done,” Leist said. “It is difficult to distinguish the extended structure in all of the JWST images, but by using deconvolution techniques, we reduced the image data to reveal the hidden faint emission feature.”
The process was also a collaboration with Willie Schaefer, UTSA’s Adobe Creative Cloud support specialist, who helped create a scientifically accurate set of color images for the study.
Leist’s work demonstrates deconvolution is an efficient and accurate tool for image processing. Similar methods, he and Packham said, can be applied to broader science cases using JWST observations. The approach has garnered significant interest from fellow scientists working on JWST image processing.
“We’re doing important work using JWST data,” Packham said. “But it’s important because we can improve on the raw data and get better image quality to see those fainter details by using this approach. It shows the strength of collaboration within the GATOS, which is co-led from UTSA.”
Leist’s work to enhance the JWST observations of the galaxy NGC 5728 is a new piece in the puzzle that further demystifies the origins of the universe. The full scope of the deconvolved images and other astrophysical results will be described in forthcoming studies currently underway by the GATOS.
“It goes back to the generation of galaxies shortly after the Big Bang,” Packham explained. “If we really want to understand our place within our own galaxy, within our own solar system and within the universe in general, we have to understand what’s going on within black holes in our galaxy and, indeed, other galaxies. We can understand the formation of our galaxy, our solar system, the earth and life on earth. It’s really part of that big picture question.”
Quelle: The University of Texas